The Discovery of Germs
The story of the explorers, experimenters, and thinkers who solved humanity's deadliest problem.
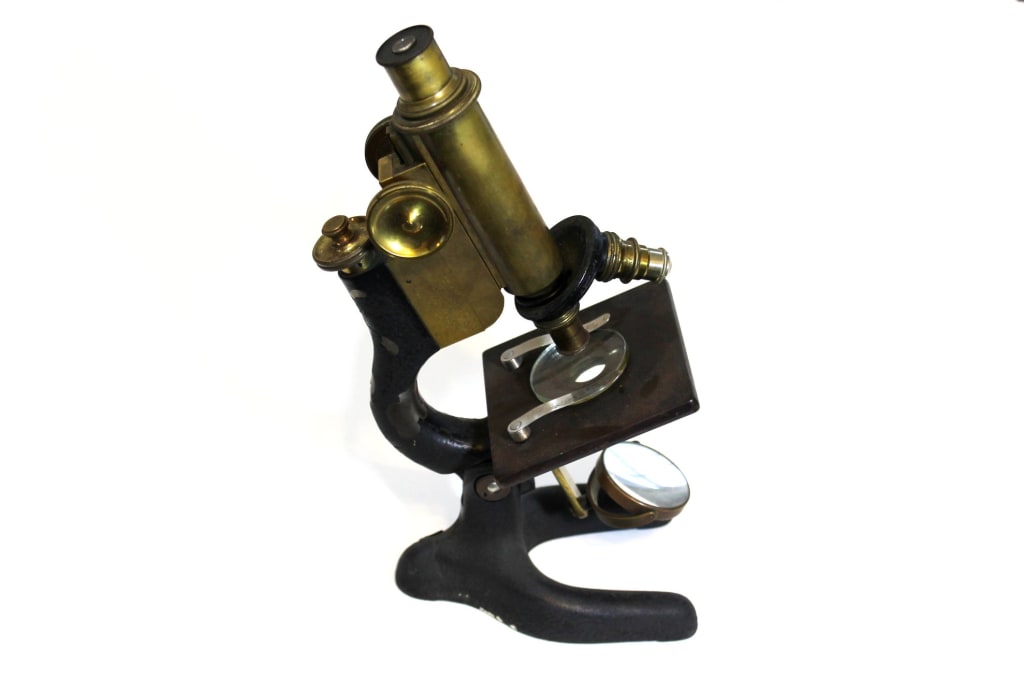
What makes you sick? Filth and decay, obviously. The stagnant muck of sewers and swamps, or even a bad basement, endangers your breathing and maybe even your life. Living in cleanliness, sunshine, and fresh air is the way to avoid damaging your health. This much has been apparent to most observers since the most primitive times. But why is it that wet things and foul places can make your inner functions go haywire? What exactly is the cause of the internal disruption? What is the reason for your dis-ease?
Sometimes the cause of a sickness might be obvious — we ate or drank something harmful, or we were bitten by a venomous creature. If the wrong substances get inside, that might make us sick. Or maybe we breathed something we shouldn’t have, something that was not fresh air. Disease has been associated with fumes and mists and stinky marshes since ancient history. (There’s even a disease named “bad air” in Latin: mal-aria.) Occasionally a particular misfortune will seem to run in the family. But there is also another possibility: maybe there are living things, like bugs, but so small that we can’t see them, and they get inside us and cause malfunctions in our internal machinery. This explanation works especially well for those deadly contagions that periodically plague mankind. The outbreaks that spread rapidly from person to person could be explained by invisible living parasites passing from host to host.
Even 1500 years before the invention of the microscope, this was not a particularly difficult guess to make. One observes infestations of worms in livestock, or one is bitten by bugs that are barely large enough to see. If there are harmful creatures barely large enough to see, maybe there are also harmful creatures even smaller than that.
But how could something invisible cause so much devastation? Tiger bites are usually more damaging than mosquito bites. Getting hit by a rock is more damaging than getting hit by a speck of dust. Even if there are creatures smaller than a speck of dust, how could they possibly be powerful enough to damage us? If they are so small our eyes can’t see them when we look right at them, and our skin can’t feel them when we touch them directly, how could they possibly cause such terrible turmoil in our lungs or our guts? Animals get sick, too, even large animals. How could something we can’t even see bring down a huge cow?
Are infectious diseases caused by invisibly small living creatures that cause disturbances in our blood or our organs, or are they caused by something else? To know for sure, in scientific terms, we need to prove a relationship between a cause and an effect. And that is not easy. Noticing a few tendencies and making a few guesses is not enough. Without exploration, experimentation, and thorough thinking, there will always be doubts and objections. The actual process of proof took centuries, and most scientists — including most doctors and biologists — doubted the existence of germs until the late 1800s. The celebrated nurse Florence Nightingale did not believe in them. What follows is a brief story of a long struggle to prove the existence of invisibly small, living agents of infectious disease. This is the story of the discovery of germs.
THE MICROSCOPE
In the year 1600, the smallest creatures in creation were gnats and fleas. Every once in awhile someone — perhaps a squinting nearsighted person, or someone with a weak magnifier — would observe tiny specks on the rind of cheese, or on someone suffering from “chronic itch”, and claim that these almost-invisible motes were actually alive. But without corroboration or any apparent significance, these claims were easily dismissed.
In the year 1600, mankind was still suffering from sporadic storms of deadly epidemics, with no clue about the true cause, and little power to evade them. London had lost over 20,000 of its residents during an outbreak of bubonic plague in 1563, and the plague was endemic in Constantinople for most of the 1500s. Neither the ancient Greeks nor any subsequent scientists had made much progress in discovering the ultimate cause of such natural disasters or in devising any kind of cure or a prevention. But 1543 was the year Copernicus published his theory about the earth circling around the sun, and in the year 1600, the Scientific Revolution has begun.
The Two-Lens Trick
If you have a transparent sphere (perhaps a glass bowl filled with water, or a glass marble), and you look through it at something on the other side, that thing appears enlarged. This effect has been known for thousands of years. “Reading stones” and magnifying glasses are just reduced versions of transparent spheres. These simple magnifiers can be fun and beautiful toys to play with. But they only make things look a few times larger at most, and for most of history they were useful mainly as an assistance, an aid to people with aging eyesight.
By the year 1600, Dutch spectacle-makers had become proficient at grinding and forming a variety of quality glass lenses, both concave and convex. One can imagine children (or adults) wandering through the workshops, playing with the lenses, and trying to see what happens when you look through two lenses at once. Many of these people probably found only blurry colors, and quickly gave up. But a few of them discovered that if you do it right, you are treated to something remarkable. Try holding a concave lens (maybe someone’s eyeglasses?) over your eye, and hold out a weak convex lens (i.e. a weak magnifying glass) in front of it. If you line them up in your sight, and look through the pair of them at something far away, they might make the far thing look closer and larger. Or look at a fly with a strong magnifying glass, then place a weak concave lens over your eye. You should be able to adjust the magnifying glass and make the fly look even larger than you could with the single lens. Using a pair of lenses in the right way, you can extend the power of a simple magnifying glass. You can upgrade a toy into an optical instrument. These discoveries were apparently made by several people independently, within a decade or so of 1600. The first written documentation is a patent application filed by Hans Lippershey in 1608, but two-lens magnifiers may have been in use as early as 1590.
In about the year 1600, a scientific giant in Italy learned of the new Dutch two-lens magnifiers. This visionary instantly saw the scientific potential of both kinds, manufactured his own optical instruments, and then used them to see things that no human eyes had ever seen before. Galileo Galilei was most interested in the ability of the “far-seeing” device to extend his eyes into outer space. He had devoted his life to proving the Copernican theory of the solar system, and he realized how important this new instrument could be in supporting his case. But he also rejoiced in the tiny wonders revealed by his “occiolino”, or “little eye”. Putting a house fly in front of his “little eye”, Galileo observed the fly’s bizarre compound eye, and he discovered how the fly could walk on walls and ceilings without falling off. He later gave one of his “occiolinos” to Federico Cesi, a young friend. This young friend admired Galileo’s new world-observing, experiment-trying spirit, and at the age of 18, founded his own “science club”. Cesi named his club the Accademia dei Lincei, using the sharp vision of the Lynx as a symbol for seeing nature clearly, and he devoted the rest of his life to his clear-seeing academy. Galileo later became a proud member, and it was members of this academy who gave Galileo’s new vision-extending instruments their modern Latin names. To the “far-seer” the Linceans gave the name “tele-scope”, and to the “small-seer” they gave the name “micro-scope”.
In the years following Galileo’s death, the telescope became very popular, and underwent rapid development. The microscope did not. The Lynx-Eyed Academy produced a few drawings of sights seen under the microscope, but sadly, it evaporated not long after the death of its founder. The microscope had not revealed new worlds, and it remained a novelty, a toy, a slightly-more-powerful magnifying glass, for decades.
The First Microscopists
Around the year 1670, the seeds of (intellectual) revolution had been planted, but in Italy at least, Authority still had fairly firm control over the “proper” views of how the world works. Galileo’s imprisonment for contradicting doctrine was still a fresh memory. But here and there, in scattered corners of Europe, a few explorers started adding lights and mountings to the microscope, started making systematic explorations with it, and started discovering new truths. At least two of these pioneers figured out how to use a microscope to look inside a body and how to perform tiny dissections, and they invented microscopic anatomy. At least two of them discovered the capillary blood vessels that link the invisible ends of arteries with the invisible ends of veins, and they removed the last significant objection to William Harvey’s recent theory that blood in a living body is constantly being recirculated. Several of these pioneers were talented artists and created beautiful color illustrations to show others what they saw. This group of five microscope-pioneers worked mostly independently but sometimes met or corresponded, and all were active at roughly the same time, from about 1660 to 1690 — two of them in the Netherlands, two in England, and one in Italy.
The Italian was Marcello Malpighi. He mapped out the anatomy of the silkworm (previously thought to be too small and simple to have internal organs), he helped discover the lymphatic system (later discovered to play an important role in the immune system), and he is the one usually given credit for discovering capillaries. The Englishmen were Nehemiah Grew, and Robert Hooke. Grew used the microscope to search for fundamental similarities between plants and animals, hoping to discover unity in God’s Creation. He found it in the realization that flowers are actually a plant’s sexual organs. Robert Hooke was primarily a physicist, but his 1665 picture-book of sights seen through the microscope was arguably the first scientific bestseller in history. Perhaps one could say it went viral. Hooke’s Micrographia included 38 plates and gave the astonished public its first view of a monster-sized gnat, a flea, and a louse, clutching a human hair. And those specks on cheese? They really are tiny bugs: cheese mites. (The specks on itchy patients are scabies mites. Those would be discovered a few years later.) Micrographia was also the inaugural publication of a brand-new science club in London: the Royal Society. This new science club included at least four of the five pioneering microscopists, as well as many of the subsequent doctors and scientists in this story, and it remains a prestigious institution today. One of the two Dutchmen was Jan Swammerdam, who performed tiny dissections and made beautiful paintings of them, and also helped to discover the lymphatic system.
Antoni van Leeuwenhoek
The remaining Dutchman was Antoni van Leeuwenhoek, and it was Leeuwenhoek who made the first major discovery on the way to understanding infectious diseases. (If you say “Loo-ven-hook”, you won’t be correct, but you’ll be close. To be more authentic, the first syllable should rhyme with pew, and the last syllable should be somewhere between hook and hoax.) Leeuwenhoek was a businessman by profession, who spent all of his free time making and using microscopes as a personal hobby. He chose a different design of microscope — a single-lens design that amounts to a very tiny but very powerful magnifying glass. He perfected the art of grinding and mounting his tiny lenses, he perfected the art of mounting specimens against the lens, and he designed a way to make delicate movements of the specimen using fine adjustment screws. (I’m not completely sure this is accurate, but I believe Leeuwenhoek can be said to have invented the movable microscope stage.) Leeuwenhoek’s hand-held microscopes were more awkward to use, but were more powerful than those of his contemporaries, and with them he observed something remarkable, something completely new, something none of the others had ever seen, or even dreamed of.
We know about Leeuwenhoek’s discoveries mainly through the numerous letters he wrote, mostly to the Royal Society of London. In one of these letters, dated 1674, he describes some beautiful, wispy, green streaks that he had observed one day in local lake water. From his description, we now know these wisps to be the lovely Spirogyra algae. And around these wisps, things were moving … not just waving in the currents, but zooming across the window, or waddling strangely, or just whirling in circles … tiny living creatures! Around the algae were minuscule little animated creatures that swam and danced and zoomed about! (Leeuwenhoek wrote exclusively in his native Dutch, and the word he used for these things is traditionally translated into English as “animalcule”. But “little animal” works as well.) Leeuwenhoek wrote: “And the motion of most of these animalcules in the water was so swift, and so various upwards, downwards and round about that ’twas wonderful to see: and I judged that some of these little creatures were above a thousand times smaller than the smallest ones I have ever yet seen upon the rind of cheese.”
Leeuwenhoek spent much of the rest of his life searching for, finding, and studying his little animals. Children delight in pets and in trips to the zoo; Leeuwenhoek delighted in the tiny zoo he had discovered inside his microscope. He searched every sample of water he could get his hands on — lakes, the sea, his roof gutters, his well, the Delft canal, drain pipes — and described new creature after new creature. Modern readers may recognize Euglena, Vorticella, Rotifers, or Hydra among his descriptions. He even found tiny little animals inside other animals — in his own mouth, for example. In one paper, he described his daily habit of cleaning his teeth, and how it left behind “a little white matter, which is as thick as if ‘twere batter … in the said matter there were very many little living animalcules, very prettily a-moving.” He provided a few sketches, and described how they “shot through the water” or “spun round like a top”. Leeuwenhoek also described the red oval corpuscles in blood and the wiggly little things in seminal fluid. To the best of my knowledge, however, he never associated any of these things with disease or parasites. Wherever he found them, “animalcules” were like the blood corpuscles: just natural residents in the place where they belonged.
How big are these little animals? They come in a range of sizes, but for some of them, Leeuwenhoek carefully compared and calculated ratios. They were astonishingly small. “This creature is a thousand times smaller than the eye of a louse!” In rough terms, these new creatures were as much smaller than lice, as lice were smaller than human beings. Where do the little animals come from? Leeuwenhoek tried an experiment. He collected some fresh rainwater straight from the sky in a thoroughly cleaned vessel, and found … nothing. But when he checked again a few days later, little animals had appeared, along with a few bits of dust and lint. He doubted that they had magically appeared from nowhere, but he was a practical man, careful never to jump to conclusions. What do the little animals eat? In a different experiment, Leeuwenhoek had prepared various “infusions” of ground pepper, ginger, cloves, nutmeg, and vinegar, hoping to discover what causes each of them to taste the way they do. Was the sharp taste of pepper due to sharp spikes or edges on the grains? After a little time, he discovered swarms of animalcules in these infusions … and thus accidentally invented microbiculture. To this day, infusions of stuff from your lawn are a great way to grow crops of microbes to put under your hobby microscope. Or to feed to your pet fish. For many years, this entire new category of life was officially named Infusoria. How does animalcule anatomy work? They have no muscles or limbs, nor any apparent brains or stomachs or hearts. Leeuwenhoek was fascinated by the organs of motion — who wouldn’t be? These bizarre creatures were clearly capable of rapid and agile motions, but they had no apparent means of propulsion or guidance. In a few of the larger creatures, Leeuwenhoek could see what looked like hair, or tentacles sticking out from somewhere, but never anything “normal” like fins or flippers.
Leeuwenhoek had discovered a new world of life … but without Robert Hooke, history might have written him off as a charlatan. Nobody could see these incredible new animalcules except through Leeuwenhoek’s personal, hand-made microscopes, and he refused to share his special techniques for making them. Some people questioned his honesty. Or competence. Or at least his eyesight. But at the request of the Royal Society, and after three attempts, Robert Hooke finally managed to produce microscopes equal to Leeuwenhoek’s. Hooke finally demonstrated to the Society that everything Leeuwenhoek had claimed was true, and he showed the rest of the world ways that they could see these things for themselves.
The Zoo Under the Microscope
Birds. Reptiles. Insects. Molluscs. The inventory of creation needed a new branch now. Leeuwenhoek had added an entirely new wing to the world’s zoological garden — the Infusoria. Now we call them more formally and more broadly the microorganisms. Or microbes, for short. The tiny occupants of this zoological wing come in all shapes and sizes, from the larger ones with a few visible parts, to the tiniest simple living shapes (often straight sticks or round spheres). And they exist everywhere we look in the natural world, living and swimming in every kind of moist matter, even inside other living bodies.
Could some of these animalcules be microscopic parasites? Could our diseases be caused by some of these microbes finding a way in and making a parasitic living inside of our bodies? But even if we found foreign microbes inside of us, how could they possibly be causing any harm? They are so undetectably small that they have no effect on our eyes when we look straight at them, and they give us no feeling or sensation when our skin touches them. How could such insensible things cause damage to our lungs or our stomachs? Surely microbes are like the flakes in a snow globe — pretty to watch, but of no practical consequence?
For almost two centuries after Leeuwenhoek’s discovery, this is how most of the world viewed microbes. They were known to exist … but they inhabited a different dimension. They were like mythical beasts, existing only in the world inside a scientist’s microscope. It was a curious world, a fun-to-visit world, but also a useless and unimportant world, of no significance outside the window.
THE EXPERIMENTAL TRADITION
What do microbes do for a living? Do they hunt? Graze? Do they make nests or hives? Where do microbes come from? Do they make seeds? Lay eggs? How do microbes work? Do they eat? Breathe? Go to the bathroom? Can microbes get sick?
Even with a microscope, there was no easy way to answer these questions. Leeuwenhoek’s microscopes were too hard to use and had very tiny “windows”. Two-lens microscopes gave broad, clear images, but only at low magnifications. So microscopists could only watch these creatures in a limited way, like a naturalist hiding in a tree and observing wildlife from afar.
What are the habits and lifestyles of the microscopic animals? Do they have any effect on us? What was needed now was more than passive observation. The way forward was to find ways to cage these microbes, to learn how to raise them, to poke them and prod them in a test tube, and to see what happens when you try different things. Learning more about the microbe world required experiments.
During the same years that the first generation of microscopists was documenting the new world inside the microscope, another intellectual descendant of Galileo branched out in a different direction. Francesco Redi was another Italian, a contemporary of Malpighi, both of them living about a generation after Galileo. Galileo’s ideas about putting the claims of authority to the test had inspired hostility from authority, but also courage and curiosity in a handful of the next generation. Redi was one of these. Redi specialized in medicine and biology, and he decided to start questioning established biological “facts” and putting them to the test. Francesco Redi was biology’s first “Mythbuster”.
Where Do Microbes Come From?
In his first work in the early 1660s, Redi cleared up some confusions about poisonous snakes, their bites, and some alleged antidotes. It turns out that eating a snake’s head does not cure you of its bite. But a few years later, in Redi’s magnum opus, he took a stand in an epic philosophical debate over the origins of life.
Where do living organisms come from? The origin of new people is obvious. Livestock, too. One could make a list of plants that obviously grow from seeds or nuts that were produced by an earlier plant. In all these cases, living things come from prior living things of the same kind. But maybe that’s only true for large, sophisticated organisms. What about swarms of smaller, cruder vermin? What about maggots, grubs, slugs, worms, ants, wasps and all other manner of squirmy, wriggly, creepy-crawly things? Many of these things do not obviously come from seeds or parents. Many of them seem to condense spontaneously out of any murky matter that can incubate them.
On the one hand, it seems nice and tidy to just assume that everything is generated in a similar way — living things might be born, hatch from eggs, or sprout from seeds, but they always come ultimately from a previous organism of the same kind. On the other hand, any devoted observer of nature could make a list of cases to the contrary. Sometimes one finds little wormy things in a place that no wormy thing entered, like maggots created in a pile of dung. Sometimes a wasp will emerge from a lump or “gall” that grew from the side of a tree. Algae and slime grow where there used to be pure water. Sometimes pesky critters seem to be born in swarms from the very ground. Wherever the ingredients come together, things get cooked up in nature’s kitchen. (At least one later philosopher tried to theorize about a living “Force” that could flow through matter and assemble the elements of life into simple living things. Maybe that’s where George Lucas got the idea.) Even worse, the assumption that all life comes from life doesn’t really solve the basic problem. To say that living things always come from parents begs the question: Where did the parents come from? It leaves you with an infinite regress. As the decades and centuries went on, and the tide of Enlightenment science rose against the bulwark of religious authority, this question would also provide an additional battlefield for religious debate. The idea that living things always come from similar ancestors fits very nicely with the orderly, religious idea of a single moment of creation; the idea that life could get cooked up here, there, anywhere, seemed like the natural, messy, godless explanation.
So which is it? Can living things arise anywhere, naturally and spontaneously, wherever conditions are right? Or must every life form have parents, even swarms and slimes of the simplest and crudest kind? Only thorough observation and experimentation could tell us for sure.
Francesco Redi believed that in at least some cases, what seemed to be spontaneous generation really wasn’t. And instead of simply asserting his belief, he tested it.
The First Controlled Experiment
Are worms generated spontaneously in putrefying meat? Redi decided to place some meat in a box, where he could watch it carefully and control what happened to it, and simply observe what happened. Sure enough, worms appeared in the meat. But then they disappeared, and Redi wasn’t sure where they had gone. So he tried again, and this time he closed the box after the worms appeared so they couldn’t get out. The trapped worms searched for a place to settle down, and then … they turned into eggs! Or at least something that looked like eggs: small, firm containers of some kind, like those made by silkworms, or by the “mealworms” you feed to your pet lizard. Wanting to observe the “eggs” separately, Redi moved them to a glass jar and waited to see what came out, if anything. They did in fact hatch … into flies! By carefully controlling the circumstances of his observation, Redi discovered that the “worms” were actually a different form of fly. Flies can transform, just like butterflies and silkworms. As we would now say, the maggot is the larva of the fly, and it goes through metamorphosis to become the adult fly. Redi repeated the test with many different kinds of meat — more than seventeen different kinds by my count, including a variety of mammals, birds, and fish. I have no idea how he obtained tiger meat. Apparently different kinds of flies prefer different kinds of meat, but in every case, Redi observed the sequence:
worm → egg → fly
Or as we would now say more formally:
larva → pupa → adult insect
But Redi still hadn’t answered the original question: Where do the worms (or larvae) come from? Redi had noticed that flies were always hovering and buzzing around the meat — usually the same kind of fly that later hatched from the worms. Was there a full circle, a “life cycle” that began and ended with the fly? Redi wrote: “Having considered these things, I began to believe that all worms found in meat were derived directly from the droppings of flies, and not from the putrefaction of meat…” Less thorough thinkers may have been content with an assumption, but not Redi. As Redi said: “Belief would be vain without the confirmation of experiment.”
But how to create a truly convincing experiment, and not just an anecdotal observation? Suppose you place fresh meat in a container on your patio, and you cover it so maggots can’t get in, and you watch what happens. If maggots fail to appear, would that really prove that they can’t appear spontaneously? Maybe the conditions weren’t right for them. Maybe you offered a kind of meat that maggots don’t like. Maybe it was too hot, or too cold, or too bright, or too dark. Maybe the maggots need air, and they couldn’t breathe in the sealed container. To really prove your case, you need to show that maggots don’t appear … but you also need to show that they would have appeared, except for the fact that you didn’t let flies in. You need to have another container, similar to your covered container in as many ways as possible, except that flies are allowed to enter one and not the other.
This was Francesco Redi’s contribution to science. This was his addition to the experimental tradition he inherited from Galileo. Redi designed the experimental control. He placed similar samples of meat in two containers, one open and one covered with gauze, allowing air to get in but not maggots. In the open container, he observed flies coming and going and the eventual appearance of maggots…which then turned into flies. In the other container, he eventually observed maggots on the outside of the gauze, trying to get in, but no maggots or flies ever entered the jar, and no maggots ever appeared in the meat. With a controlled experiment, Redi had shown that maggots were not generated spontaneously in rotting meat. They were deposited there by flies.
A few of Redi’s followers tried similar experiments and expanded Redi’s conclusions to other insects. The microscopists also discovered a few examples of insects laying eggs that nobody had noticed before because the eggs were too small to see without the microscope. In his later work on parasites, Redi himself discovered numerous examples of invisibly small eggs.
Redi and his contemporaries had discovered many examples of invisible eggs and of metamorphosis of worms into bugs. But had they proven that all life must come from prior life? Even Redi didn’t believe it was true as a universal principle. He was unable to find an explanation for the appearance of gall flies and intestinal worms, for example, and reluctantly concluded that these were indeed spontaneously generated.
Incidentally, one of Redi’s younger associates was a doctor named Giovanni Cosimo Bonomo. Most microscopists up until now had been professional scientists or microscope hobbyists. Bonomo was one of the first professional doctors to try to see if the microscope had any value in the practice of medicine. One day, he poked loose some material from the skin of a chronically itchy patient, trained his microscope on the ooze that came from it, and discovered tiny creatures. They had numerous legs, and “two little horns at the end of a snout”. Redi’s friend Bonomo was the discoverer of the scabies mite. This discovery could be considered the first reasonable evidence for a link between a disease and a microscopic cause … but it was hardly conclusive. Bonomo had a big row with the church over it, and the idea the the mite causes the itch was pretty well rejected until the time of Pasteur. As with Leeuwenhoek’s little animals, most people thought that the scabies mite was just a harmless tiny thing seeking its natural habitat.
The First Peer-Review
Now what about microbes? Even if all “normal” life comes from eggs or seeds or parents, surely the same does not apply to the weird, tiny, rudimentary aliens in the microscope? Maybe insects count as complex “higher” organisms, but surely the tiniest, crudest forms of life get brewed up wherever there is a fertile infusion for them to swim in? We never see microbes lay eggs or give birth, like normal animals. They just appear or they don’t.
So are microscopic animals cooked up spontaneously or not? Again, only careful observation and experimentation could tell us for sure. Leeuwenhoek had performed an experiment or two with his little animals, and he was reluctant to believe in spontaneous generation even for microbes, but he was an explorer in an uncharted realm, and he was admirably cautious about jumping to conclusions. More extensive testing was needed.
In 1748, an English Roman Catholic priest by the name of John Turberville Needham read about animalcules. He wanted to see if they could appear from nothing, so he took a trip to his kitchen. He cooked some gravy over a fire, poured it into a bottle and corked it, and then waited. After a few days, he opened the container, placed some of the contents under a microscope, and saw … animalcules! He had offered nature a nurturing and sterile medium, and nature had brewed a fresh crop of microbes inside.
About two decades later, an Italian Roman Catholic priest by the name of Lazzaro Spallanzani caught wind of Needham’s experiment. And something smelled fishy. Spallanzani knew about Redi’s work, and he admired Redi’s carefulness and experimental technique. In contrast, Needham’s experiment sounded sloppy. How long had Needham cooked the gravy? Had he adequately sealed the container? Microbes are pretty tiny — maybe they had found a way to get in through the cracks around the cork. Spallanzani conducted his own set of tests, this time with a variety of sealing methods and a variety of cooking times as comparisons and controls. Spallanzani picked up the tradition that Galileo had started and Redi had built upon, and he continued the war for truth by experience and experiment.
In some tests, Spallanzani poured the gravy into a flask with a glass neck, and then used a flame to melt the neck, fusing the glass itself over the opening and making a truly impermeable airtight container. These impenetrable flasks he boiled for an hour, and then he allowed them to stand for several days. When he broke them open and examined the contents, he found nothing but sterile gravy. Nature had not been able to form any microbes in those perfectly sealed containers. In another series of tests, he simply popped a cork into the mouth of the flask. He boiled these for an hour also, to ensure they were sterile to begin with, then waited. This time, the gravy teemed with life. Needham had apparently been sloppy, and allowed microbes to get in around the cork. Spallanzani showed that if you really seal a sterile flask, then no microbes will appear inside.
Needham did not take Spallanzani’s rebuttal lying down. More experimental dueling ensued, but I think our story can pass over it. To this day, “peer review” is a valuable component of the self-correcting mechanism of scientific discovery, but it runs the risk of becoming personal and contentious at times.
Where DO Microbes Come From?
If microbes cannot appear from nothing, then where do they come from? When you see two normal animals stuck together they are probably mating. Sometimes you see two microbes stuck together. Are they mating? Spallanzani didn’t immediately attack this question himself — he was a busy man with diverse scientific interests — but his puzzle found its way to a fellow Renaissance Man in nearby Geneva. Horace Bénédict de Saussure attempted to corral some microbes in an infusion of hemp seed and watch them closely, and he eventually came to the opposite conclusion. Each doublet was not two animals coming together … but one animal splitting apart! Microbes seem to have their own way of doing things in every area of life: eating, moving around … and reproducing. Perhaps their unique way of reproducing is not by laying eggs or giving birth, but simply by splitting into two halves.
Or maybe splitting is just a rare accident. Maybe every once in awhile, one of the speedy microbes comes zooming along without watching where it is going, and it smacks into another one, and breaks it into two pieces. That was the idea of John Ellis, who repeated de Saussure’s experiments and observed that only a small percentage of microbes divide. He also insisted that the normal way for microbes to reproduce is by giving birth, because he had seen children microbes inside parent microbes, and even grandchildren microbes inside the children microbes.
Spallanzani heard about de Saussure’s observation, and about Ellis’ theory, and again something smelled fishy. He decided to focus his attention back onto microbes, and to show once more what thorough, careful experimentation can do. He placed a droplet of infusion, bustling with microbes, on a piece of glass, next to a droplet of pure water. With a delicate probe, he teased out a fine bridge of water connecting the two droplets, then carefully watched and waited. Eventually a rambling microbe wandered curiously across the bridge. Spallanzani quickly wiped away the bridge, trapping the microbe in a pond by itself. Spallanzani had invented a way to isolate a single microbe. He watched this loner carefully … and eventually he saw it split into two. Then he watched the pieces split again. He repeated the experiment and watched the division many times. For microbes, this was not a rare event, caused by accident. Reproduction by division is routine procedure.
The Intellectual Tradition
Where do new microbes come from? That question now had an observable answer, a demonstrable alternative to spontaneous generation. But there was still the question of where the parent microbes came from, and it had not yet been proven that microbes cannot self-assemble. Maybe they can generate in more than one way. After Spallanzani, the debate over spontaneous generation would flare up one final time, before being laid more or less permanently to rest.
I think you could argue that there is one living thing that is spontaneously generated … and that is an idea. Ideas and philosophies can grow and evolve from their predecessors like any living thing. But every original idea has to appear for the first time somewhere. It has to be born in the mind of a creative thinker, by an act of will. If there is some kind of “force” capable of assembling prior elements into new and unique creations, that force is free will. Human will is what arranges observations and prior ideas into new ideas. The knowledge of humanity grows by addition, each new thought being created and integrated at some place in the world and some point in history, by the effort of some one man’s mind. I am not a historian and I don’t know how much credit to give to Renaissance thinkers prior to Galileo. But when recognizing giants of originality, Galileo must be in the first ranking. In this one man was born a philosophy that you should look with your own eyes, put two and two together for yourself, and test the assertions of authority by experience and experiment. And this intellectual bonfire ignited chains of sparks that can be traced through all of subsequent intellectual history. In biology, Galileo ignited a tradition that passed to Francesco Redi, the Mythbuster who invented the controlled experiment, and then to Spallanzani, the man who cleaned up other people’s messes and demonstrated the origin of invisible things.
The illuminating torch of careful experimentation passed from Galileo to Redi to Spallanzani … and then it passed across the Alps from Italy to France. It passed to a successor who gratefully hung Spallanzani’s portrait on his wall, improved Spallanzani’s experiments, and showed how microbes move the world. This man showed that microbes alter nature, and can sometimes alter health. He also delivered the final coup de grâce to the theory of spontaneous generation of germs, enabling us to defend ourselves against them. This man was Louis Pasteur.
The Next Generation of Microscopes
In the 18th century, the world had more or less given up on Leeuwenhoek’s single-lens design, because it was inconvenient to use and it was hard on the eyes. To get the best results, one had to have sharp natural eyesight (which Leeuwenhoek probably had). On the other hand, the simple two-lens design started to show colored fringes above a magnification of 30 or 40 or so. In effect, when you demanded too much strength from the lenses, they started to act like prisms and turned the images into wet watercolor paintings. The simple two-lens microscopes were useless at high power.
1824, an English wine-seller and natural history enthusiast named J.J. Lister decided to see if he could make each lens out of two different kinds of glass, the second one correcting the mistakes of the first. He succeeded, and invented the first achromatic lens. These do not act like prisms, even at high magnifications. With a pair of achromatic lenses, two-lens microscopes could give comfortable, roomy viewing windows with crystal-clear images, even at magnifications well beyond those of Leeuwenhoek’s microscopes. Thanks to J.J. Lister, the next generation of microscopes was born, and the optical microscope took on the modern design that we know today. These modern microscopes could now make out structures (were they internal organs?) inside the larger animalcules, and they could give a much better look at the tiniest of the animalcules, such as the ones Leeuwenhoek had found in his teeth. In the ocean of whales and minnows, we could finally get a good look at the minnows.
By 1850, with the aid of the improved microscopes, the list of different kinds of microbes had expanded into a catalog, organized crudely into categories by size and shape. In Pasteur’s day, grouping and naming microbe families was still a messy business, but there were a few broad categories. There were the huge “whales” of the microbe world with visible internal organs — these were the protozoans. There were also swarms or “colonies” of tiny rods (bacilli), tiny spheres (micrococcus), and tiny spirals (spirillum and spirochaeta). Modern readers may be familiar with the names streptococcus and staphylococcus — those are tiny spheres that grow in long chains resembling chains of beads, and tiny spheres that grow in clusters resembling clumps of grapes. Collectively, all these tiny “minnows” with simple shapes were the bacteria.
In 1850, armed with a heritage of careful experimentation and a new generation of microscopes, Louis Pasteur was about to show for the first time what the microbes could do.
The Lives of Microbes
In the early 1850s, Louis Pasteur finished his education in physics and chemistry, and began to climb the ranks of French Academia. In 1856, French sugar-beet growers were having problems with their ferments, and sought advice from science. (Apparently sugar beets are good for making rum.) They enlisted young Pasteur to attack their problem. Fermentation was understood to be a chemical process that converts sugar into alcohol, and who better than a chemist to study what happens when fermentation goes wrong?
Wine and Milk
To ferment a batch of alcohol, the beet growers would honor their traditions, follow their recipes, boil their beets, and mix up their mashes in the prescribed way in the prescribed containers. And they were usually rewarded by a batch of fermented beetroot alcohol. But sometimes the ferment would go bad, and for no apparent reason the distillers would end up with a batch of sour juice instead of the desired alcohol.
Pasteur, trained as a chemist, easily discovered what was making the bad batches taste sour. They contained the acid of sour milk. Or as chemists say now: lactic acid. But where had it come from? Somehow there had been a different conversion process — one that produced lactic acid when it should have produced alcohol.
What was the cause of the different outcomes? Pasteur decided to see if he could find any interesting differences under the microscope. From a successful ferment, he scooped up a little of the froth, and placed it under the lens of microscope. When the picture came into focus, he saw … not animalcules, but something new. There were weird globules, just sitting there. In the microbic ocean of whales and minnows these oval globs were quite small, but still a few times larger than the minnows. Pasteur had discovered a new globular kind of microorganism a little larger than bacteria. He had discovered the yeasts.
Actually, he had re-discovered the yeasts. In 1835, the French physicist Charles Cagniard de la Tour had examined brewer’s yeast under a microscope, and observed the globules. He had even realized that the globules were alive, because he saw them reproduce … but they reproduced in a weird new way. When a microbe reproduces by division, it pinches itself across the middle to form two equal halves. Cagniard de la Tour watched tiny little nubs start on one side the yeasts, grow larger and larger, and eventually detach themselves. Cagniard de la Tour had discovered that yeasts reproduce by budding. And this reproduction of the living yeast probably had something to do with the process of fermentation … but what?
In his good ferments Pasteur re-discovered the yeasts, and the budding, and then naturally wondered what he would find in the bad ferments. From a sour ferment, he scooped up a little of the froth, and placed it under the lens. When the picture came into focus, he saw … tiny rod-shaped things, even smaller than the yeasts. Were they a new kind of living bacilli?
Pasteur realized that he needed to try to grow them. If they were living bacteria, he should be able to find a healthy soup that they could live in, and then raise a crop in the laboratory. But he also needed a clear soup, so he could watch them while they grew and lived. He tried pure sugar-water, but the rods didn’t like that. Eventually he came up with a recipe for a clear broth that made the strange rods grow. Whenever he introduced the rods into the broth, they always multiplied, and the more they multiplied, the more acid of sour milk appeared. Not only were the rods alive, they were manufacturing the lactic acid as they grew!
Why did they make acid? Did it serve a defensive purpose, or was it simply a waste product, the microbe equivalent of urine? In any case, the lactic acid was coming from the bacilli, as a natural part of their living processes … just as the alcohol was probably coming from the living yeasts, as a natural part of their living processes. Wherever there were yeasts, there was alcohol, growing in quantity as the yeasts reproduced. And wherever there were these bacilli, there was the acid of sour milk, increasing in quantity as the bacilli reproduced.
By isolating the bacilli, figuring out how to culture them, and studying them separately in their own test tube, Pasteur had shown for the first time in history that microbes are meaningful. By their collective actions, they could alter the world. Now we know Pasteur’s rods by the name lactobacilli, and lactic acid fermentation is their lifestyle. They are an important component of the human microbiome, and are used to ferment foods from kimchi to yogurt.
But there were still exceptions. Sometimes Pasteur would attempt to grow the bacilli and perform lactic acid fermentation on purpose. (It was a little like making sauerkraut, except in a test tube.) But sometimes instead of sour acid there was the smell of rancid butter. Was this yet another kind of “fermentation”? When Pasteur examined this new smelly “ferment” under the microscope, he found a new kind of microbe. Three different chemical products, three different microbes, three kinds of “fermentation”.
And then the global power of microbes must have started to come together in Pasteur’s mind. The souring of milk is a kind of fermentation, similar to alcohol fermentation…and also similar to putrefaction, the smelly decay of organic matter. And living microscopic organisms are responsible for all three conversions. Maybe all fermentation, all putrefaction, and all decomposition of any organic matter, is the work of microbes simply going about their normal lives. Microbes don’t alter the world just a little bit, they terraform the globe. In all the world, in all the soil, in the corpses of every plant and animal that nature has ever produced, some chemical agent must be responsible for “digesting” that matter, for causing it to decay, for reducing it to raw ingredients, for creating the soil from which the next generation of life can emerge. If this did not happen, new life would not be possible. The globe would be a landfill packed with every dead plant and animal that ever existed. Something recycles the matter from every generation of life and prepares the nutrients for the next generation. That something is microbes, and Pasteur’s vision saw it for the first time.
And what about disease? If microbes can transform the soil of the world, maybe they can transform our insides, too? Maybe they weren’t so innocent and blameless of disease after all. In Pasteur’s time there had been growing incidence of various smelly, colorful, pus-producing and fever-inducing “hospital diseases”, like gangrene, erysipelas, and pyaemia. There was also a growing recognition that these smelly diseases of decay bore a certain resemblance to putrefaction. Maybe these “diseases of putrefaction” were caused by microbes-of-putrefaction? Maybe these microbes get in through open wounds or surgical incisions and try to decompose our insides?
But where were all these microbes coming from? When there is a fresh wound, or an open container of sterile beetroot mash, the microbes-of-putrefaction or the microbes-of-fermentation just appear without being added. How did they get there? This brings us to the final eruption of the spontaneous generation debate, in which Louis Pasteur finally settled the issue of where microbes come from.
The Swan-Neck Flasks
Pasteur, like Redi and Spallanzani, did not believe that microbes could appear without parents. But if that is true, then the only explanation for the appearance of microbes in places where there were no microbes before, is that they can fly. Well, maybe not fly. But maybe they can drift through the air like motes of dust? Most microbes are swimmers — can they stand drying out, and travel alone through the air? If not, maybe they can hitch a ride in a droplet of mist, or on a damp particle of dust?
Pasteur wanted to show once and for all that microbes cannot come from nothing. They must enter new areas by traveling through the air. But now Pasteur had a problem similar to Francesco Redi’s. If he simply sealed a bottle and sterilized it, and no microbes showed up, would that really prove that they can’t be generated spontaneously? His opponents could argue that the sterilization damaged the air somehow, or that the microbes need fresh air to breathe. Pasteur needed to figure out a way to allow fresh air to get into the bottle, but to keep dust out.
Pasteur’s solution? The famous swan-neck flask. The broth would rest in a glass globe equipped with a long, narrow neck. The fine neck would allow air to pass through, but would prevent wind from blowing dust or debris into the interior. To prevent dust from falling down the neck under gravity, the neck arced up and over and back down again. Dust would have to fall up to reach the interior of the flask. Fresh air could pass in and out through the long narrow twisty neck, but no dust could blow or fall in.
Pasteur boiled his broth in the fancy flask, and then he waited. After several days, despite the fact that the rich nourishing broth had been exposed to open air, there were no microbes. In the spirit of Francesco Redi’s controlled experiments, Pasteur then carefully broke off the curved portion of the neck allowing dust to fall in through the newly exposed opening in the top of the flask. A few days later, the broth was full of microbes. Pasteur had finally shown how new microbes can appear in formerly sterile places. They can ride in with the dust in the air.
Pasteur even developed his own method for testing the air in different places to see how many and what kinds of microbes it contained. It was a sort of low-tech air purity tester. He prepared many sealed sterile flasks with broth. He sealed them by fusing the glass, as Spallanzani had done, and he did it while the broth was at full boil, so that there would be a vacuum inside when it cooled down again. These flasks were his air testers. He would take them into whichever air he wished to test, then break the necks. Air would rush in to fill the vacuum, and then Pasteur would simply re-seal them, and incubate them. If the air had been full of microbes, the broth would soon turn cloudy. If the air had been clean, the broth would remain clear. He used such flasks to show that the air outdoors was full of microbes, that the air in a clean and calm storage vault was relatively microbe-free, and that the higher he climbed on a mountain the fewer microbes there were in the air.
Antiseptic Surgery
J.J. Lister, the man who invented the achromatic lens and revolutionized the microscope, had a son who revolutionized surgery.
As a child, Joseph Lister absorbed his father’s interest in natural history and microscopes. Then he grew up and became a professor of surgery at the University of Glasgow. Like many doctors of the time, he lamented the state of his own profession. Smelly “hospital diseases” like gangrene and pyaemia were widespread, and half of all surgeries ended in death. Then Lister read about Pasteur’s work with microbes. If hospital diseases were being caused by living airborne germs, then preventing them should be a matter of keeping the air and the surgical instruments sterile, and killing any germs that were already in the wound. Killing microbes is easy. But how in the world do you kill the microbes in a wound without killing the patient?
In 1865, after searching for a suitable “anti-septic”, Lister settled on a substance now called phenol, and he developed a careful procedure for swabbing wounds with this disinfectant. His ideas and techniques were mocked at first, but in a few years, his surgical death rate dropped from 45% to 15%. A decade later, the rate was down to 5%. Joseph Lister saved countless lives, saved limbs from amputation, and saved the King from appendicitis. He was honored with titles, medals, and statues from several nations, and his name is now immortalized in medicine cabinets everywhere on the label of a popular green mouthwash.
The Diseases of Silkworms
While Lister was working out his new procedure for antiseptic surgery, the French silk industry was suffering from problems of its own. Silkworms were being ruined by the millions by disease, and the silk industry’s revenue had plummeted to a tiny fraction of what it once was. In 1865, the French Minister of Agriculture asked Pasteur to turn his attentions to the new problem.
Unfortunately, the diseases of French silkworms were a little like the Gordian Knot. Silkworms suffer from multiple diseases with different causes, and at least two of them were active in Pasteur’s time. The diseases also affect the eggs, the worms, and the adult moths differently. Pasteur never did untangle the knot and conclusively link a single specific disease with a single identifiable microbe. But like Alexander, Pasteur found a way to cut through the knot and move on. With his microscope and his experiments, Pasteur showed that the diseases were transmissible and were due to living agents spreading from worm to worm. Even if he didn’t know for sure which living agents caused which disease, he at least figured out a way to identify which creatures were sick and keep them from infecting the healthy ones. The following year, the growers enjoyed a splendid yield of silk, and life in the French silk industry could begin to get back to normal.
The Germ Theory
Before Louis Pasteur, microbes were curiosities that existed only inside a scientist’s microscope. Pasteur showed that microbes affect and alter the world. They consume and emit materials just like any other living thing, and their collective chemical processing is constantly reshaping the earth. Microbes are not like flakes in a snow globe. They are like flakes of snow — each one may be insignificant, but collectively they can change the world. Pasteur also showed us that different microbes do different things. They are not random chaotic clowns of nature. Each kind of microbe has its own anatomy, its own habits, its own needs, and its own effects, just like any other living species.
The microscope revealed the world of microbes. Better microscopes and careful experimentation revealed the diversity and the significance of this world. The world of microscopic living organisms was now known to exist, and the habits and lifestyles of the microbes were coming into focus.
But do microbes actually cause human epidemics? Are the outbreaks that plague humanity due to microscopic pests, passing from host to host? Microbes cause fermentation and putrefaction, including the “diseases of putrefaction” that appear in unsanitary hospitals. And some kinds of microbes seem to be responsible for the mess of illnesses that appear in silkworms. It was not a huge leap to assume that infectious human diseases are caused by parasitic microorganisms, each disease by its own “seed” or “germ”. Pasteur had also shown that even microbe parasites cannot appear spontaneously inside a host. They can only harm a person if they can find a passageway in from the outside. And if that was true, then mankind finally had a way to attack its deadliest problem. Mankind finally had a weapon against the curse of epidemics and plagues.
Pasteur became convinced of his “germ theory” and became a crusader against germs. He delivered passionate lectures to his students on the subject, and announced to the public: “It is in the power of man to make parasitic maladies disappear from the face of the globe, if the doctrine of spontaneous generation is wrong, as I am sure it is.”
But it was still an assumption. There still had been no conclusive proof and no definitive link between human epidemics and microscopic organisms. There was still no knowledge of specific causes and no way to diagnose specific diseases, much less cure them.
By some accounts, Pasteur could be prone to streaks of arrogance and pettiness. The Franco-Prussian War apparently turned him against all things Prussian, and following his success with the silkworms, he turned his attention to a revenge project: making French beer better than German beer.
It may also have been his national pride that turned Pasteur back to the problem of human diseases. After seeing the success of a disease-hunting German, Pasteur re-dedicated himself to the hunting of germs and the extermination of disease. He would eventually provide mankind with weapons of mass destruction against several deadly diseases, and by showing how such vaccines could be made, he would inspire the creation of many more. In the saving of lives from deadly diseases, Pasteur would become a giant … but only after someone else first proved the cause. For the time being, our story crosses the Rhine and passes from France into Germany. We turn to the careful, patient, clarity-producing, self-made scientist who linked specific epidemic diseases with specific microbial causes for the first time, and made germ-hunting into a science.
CAUSE AND EFFECT
I think Hercule Poirot would have admired Robert Koch. With rigorous “order and method,” Koch was the man who finally proved beyond a reasonable doubt the guilt of the culprits behind three crimes against humanity. Koch was the man who finally convicted the first three germs of disease.
Koch, the Microscope, and Splenic Fever
In the late 1860s, a young Robert Koch graduated from a German medical school, repaired some soldiers during the Franco-Prussian war, married, and then settled down to a quiet life as a respectable country doctor. In 1871, on his 28th birthday, Frau Koch bought her husband a microscope to play with and inadvertently launched his career as a germ detective.
While Koch played and practiced with his new microscope, the sheep of Europe were dying by the flock from a mysterious ailment that would turn their blood and their spleens black. The spleens would swell with this blackened blood until they almost seemed to fill the torso. Occasionally shepherds would get feverish, too, and break out in black sores. Sometimes they would die. Certain fields and pastures of Europe had become deadly ground — any flocks of sheep or herds of cattle that grazed upon these particular cursed lands would become feverish and die with blackened blood and swollen spleens, even if the fields had been untouched for years. Was there something different in the local soil? Something wrong with the local water supply? The local weather? This particular mysterious affliction went by a variety of names: splenic fever, the black disease, or in France le charbon, the charcoal disease. Now we call it anthrax.
As he attended to his patients with one disease or another, Doctor Koch would sometimes despair over his ignorance and his inability to do anything but dispense empty words and palliative pills. Even in Koch’s day and age, doctors still didn’t understand what really caused all that interference with people’s breathing or digestion or blood flow. And without understanding, there was little they could do. It was very disheartening. But in his newfound hobby as a microscopist, Koch was growing in skill and technique, and would put whatever he could find under the lens. So when anthrax broke out in the countryside around him, it was only natural that he would turn his microscope onto the blood of anthracitic sheep … and rediscover strange rod-shaped things existing there. A few other doctors-with-microscopes had already noticed “stick-shaped corpuscles” in the blood of anthrax victims. These sticks looked somewhat like the blood corpuscles (nowadays we call them red blood cells), but these new intruders were rod-shaped and slightly smaller (nowadays we call them bacilli). Often they would line up end-to-end in long winding chains, vaguely resembling a freight train. They might make one think of infusoria except these were found in mammalian blood instead of in water, and they didn’t wiggle, or move, or grow, or change in any way that would show they were alive. The doctors-with-microscopes before Koch had even guessed that these sticks might have something to do with the disease. Maybe they were a consequence of the disease. Or maybe they were even the cause. That guess, however, was to most educated people of the time, a baseless and foolish notion. How could something so invisibly tiny cause enough harm to kill a huge cow? Probably those sticks-in-the-blood were just some kind of bodily residue, some debris of the disease, or at worst, harmless colonists simply seeking diseased blood as their natural habitat. They surely weren’t the cause.
Koch saw these sticks. Koch suspected they might be the cause of the disease. Then Koch set himself to the challenge of proving it, one way or the other.
Anthrax
If these strange bacilli were the true “seeds” or “germs” of anthrax, if their presence was the cause of the fever, blackness, and death, then these bacilli should always be present in the blood of diseased animals, and they should never be found in healthy animals. So Koch began to gather his evidence by searching the blood of animals — hundreds of them — and passing sample, after sample, after sample, under his lens. In all blood samples from diseased animals, as long as the disease had progressed sufficiently to show symptoms, he usually found the strange trains of rods in the blood. In healthy animals, he never did. So far, so good. These bacilli definitely seemed to be associated with the disease somehow.
Next, if these bacilli were the cause of anthrax, then they should turn a healthy animal into a sick one after they entered the animal for the first time. Koch needed to show that the change from a healthy animal to a sick one happened when there was one and only one other change: the entry of the bacilli into the animal’s body. Also, there has been no evidence yet that these sticks were actually living things. If those quiet sticks in the microscope field were in fact living parasitic germs, capable of growing and reproducing in their host, it would be nice to be able to at least show that these sticks could multiply.
But here Koch faced problems. All of these things demanded experiments. Preferably a great many of experiments. Putting together the rest of the puzzle and building the rest of the case against the germ was going to require lab work. But there was nobody to teach Koch how to do it. Apart from Pasteur, almost nobody in the world knew the first thing about measuring or nurturing microbes. So Koch single-handedly developed the art of handling diseases in a lab.
Lab Work
First of all, filling a laboratory with messy, noisy, hungry, diseased sheep was out of the question. Mice would be much better. But can mice be given anthrax? And how, for Pete’s sake? Maybe one could poke a little diseased sheep blood into them? But then how could you be sure you weren’t contaminating the mice with any other microbes from around the laboratory? (The hollow hypodermic needle had been invented a couple of decades earlier, but I’m not sure if Koch knew of it, or if he could obtain sufficient quantities cheaply.) With practice, Koch eventually worked out a reliable procedure for sterilizing wooden splinters, dipping them in anthracitic blood, and poking them into mice. The mice fell ill, and died. Many mice, consistently. But had the mice actually died from anthrax, or from something else? Koch became an expert at murine autopsies. Sure enough, in the deceased mice, the blood and spleen were always black. What about the bacilli? Under the microscope went samples of blood and spleen. There were the sticks-in-the-blood. Koch had invented a way to give anthrax to mice artificially in a laboratory, by contaminating them with diseased blood. He had found a useful experimental animal, and had shown that the disease can be transmitted by something living, or at least reproducing, in the blood. And as always, he continued to find the mystery sticks in all diseased blood, and never in healthy blood.
So the case for the prosecution was getting stronger. But the defense still had reasonable doubt. The bacilli had not been shown to have any actual activity or measurable effect, nor even to be alive.
If these sticks-in-the-blood were living organisms, then perhaps it would be possible to culture them, to grow crops of them separately in their own “germ aquarium” of some kind. And perhaps if one could do that, then one could watch them eat and grow and reproduce? One could watch them and handle them in their own test tubes, rather than having to work inside a living sheep or mouse. One could observe and experiment in vitro, rather than in vivo.
But how? If the bacilli are alive, what do they like to eat? Do you need to keep them warm? Koch reasoned that, if they thrive in mammalian bodies, they probably prefer to be kept warm, and they might like to live in mammalian fluids. But he also needed to keep them in a clear fluid, so he could watch them.
Anyone who has ever taught or participated in a middle-school science class may be familiar with cow eyeballs. They are readily available as a by-product of beef production and are relatively easy and fun to dissect. If you have ever dissected one, you may remember the watery fluid that leaks out when you cut into the cornea. This is the aqueous humor, and Koch realized that it might be just the clear, nutritious incubation fluid he had been looking for. So he jury-rigged a body-temperature incubator, placed a small piece of diseased mouse spleen into cow eyeball juice, placed this test sample into the incubator, and waited.
His incubator worked too well. If there were any anthrax-sticks in his culture, they were completely hidden by the jungle of other microbes that had overtaken his microbe-aquarium. Apparently, microbes had traveled in from harsher conditions, thrived in the luxury environment Koch had set up for them, and crowded out the anthrax-sticks. Now what to do? Koch needed to find a way to block out microbes from the surroundings.
Perhaps Koch could have used swan-neck flasks like Pasteur, but those wouldn’t have made it very convenient to work with the microbes. Instead, Koch invented another very simple, ingenious solution. He placed a tiny shred of mouse-spleen in a droplet of cow-eyeball-juice onto a thin glass slide, then placed the slide upside down over another piece of glass with a little hollow well formed into it. The droplet hung there, in its own closed chamber between two pieces of glass, cut off from the outside world, out of the reach of dust or any other microbes.
This time, after incubation, the jungle contained only one species: the anthrax bacilli. Over the duration of a few hours, they had grown from a few threads clinging on to the bits of spleen, to massive tangles of twine surrounding them. They still didn’t appear to wiggle or breathe or grow, but in those few hours they must have multiplied because now there were many where once there were few. They had multiplied like a living thing. Koch had known they must, because a few of them on a wooden splinter could turn into a mouse-full a day later. But now he had clearly demonstrated the existence of reproduction outside the mouse under controlled, pure, laboratory conditions, for the first time.
Would this new generation of bacilli cause anthrax? Koch extracted a small sample, and placed it, not into a mouse, but into another hanging-drop culture-well. He incubated this, and then repeated the process several more times, starting each new culture from a carefully chosen sample of the previous one. He wanted to be sure he had a pure-bred culture of anthrax bacilli, with no trace of contamination from anything else. Finally after eight generations, he found himself with a purified culture of anthrax bacilli that hadn’t seen living animal flesh since their ancient ancestors, and with these far descendants he inoculated a mouse. It fell ill, and died. Again Koch confirmed by autopsy that it had black blood and a swollen spleen. Again he confirmed by microscope that the bacilli filled the blackened blood.
The case for the prosecution was now pretty convincing. These tiny rod-like bacilli existed in all mice killed by anthrax and were never found in healthy mice. They could reproduce and be cultured, and their introduction into a healthy mouse was followed by death by anthrax. For the first time in history, Koch had made certain that one particular kind of microbe was the cause of one particular kind of disease.
But maybe mice are different from sheep? Koch now had reliable and well-practiced procedures, and he methodically repeated his disease-and-germ tests with guinea pigs, rabbits, and eventually sheep. He always found the same thing.
The Mystery of the Cursed Fields
Were anthrax bacilli the cause of the anthrax disease? Almost surely. But one credible rebuttal still remained: the cursed fields.
We can suppose that the living germs of anthrax, like most living parasites, leave their host at some point and find a new one. Perhaps we could explain the cursed fields by supposing they are full of anthrax bacilli, deposited there by their previous host and lying in wait for the next host. But Koch’s germs, if they were in fact living things, could hardly be immortal or invulnerable. They must die without food or in hostile conditions. Koch himself had observed his cultures to weaken and disappear when neglected too long or when kept in the wrong conditions. If the natural environment of these anthrax bacilli was the warm, rich, comfortable blood of mammals, then how in the world could one explain the existence of germs in a cold mountain field, which had been free of livestock for years and subjected to frosts and droughts and who knows what else in the meantime? What kept the germs alive in fields and mountains season after season when they died on glass in two days?
Koch found the answer, and his final re-rebuttal, in one of his failed, dried-up cultures. In the samples of faded and apparently dead germs, he noticed that the former culture had filled with little oval speckles. Was this contamination by some other interloping microbe? Closer inspection revealed that the speckles were actually inside the corpses of the former germs. Was it some sort of decomposition? Did the bacilli disintegrate into nuggets when they died? Koch kept that sample for a month, and noticed no change. Then he decided to see what would happen if he put fresh cow eyeball juice on these speckles. Would they dissolve? Would they grow and reproduce into clouds of speckles? No, they turned back into long chains of anthrax rods! Could these regenerated bacilli cause anthrax, like the original pre-speckle bacilli? Yes, they could. Koch performed more experiments, and found that these speckles could weather and wait out cold, heat (up to a point), and drying out, and they could remain infectious for months under a range of adverse conditions. The anthrax bacilli had a trick up their sleeves, and Koch had just found them out. Whenever times were lean or conditions were harsh, the bacilli could package themselves for storage, into these hard, durable, inactive, hibernating speckles, and the speckles could unpack themselves back into living bacilli whenever they were returned to healthy (for the bacilli) conditions. Koch had discovered that some bacteria can form spores.
So much for the mystery of the cursed fields. They were not cursed, they were full of spores of anthrax bacilli.
The Public Indictment
The year was 1876. Koch still couldn’t name the mechanism by which the tiny germ caused wholesale physiological disruption to the giant it inhabited. He couldn’t explain how the habits of the germ made spleens swell or blood turn black. But Koch finally felt confident enough to make a public indictment. He knew that these bacilli were the cause of the disease anthrax. He packed his bags with samples and equipment, rode a train to the University of Breslau, and delivered a three-day lecture, complete with demonstrations, illustrations, and plenty of microscopes. He presented his case thoroughly and methodically, with overwhelming evidence and unassailable logic. He won over the audience, and the scientific community at large. The doctor and self-made scientist had expertly proven a cause and effect relationship. For the first time in history, Koch had securely linked an infectious disease to a microbial cause — the germ now known as Bacillus anthracis.
Human Diseases
Anthrax is mostly a plague upon livestock, on herbivorous mammals and maybe rodents. Human beings can catch it from animals, but it doesn’t usually spread from person to person, nor cause epidemics among populations. It is mostly a concern of individuals who work with livestock, or people who travel to strange lands.
If you lived in Koch’s day, you were probably far more worried about the chronic, spitting, bloody cough that was slowing consuming people from the inside out across much of Europe. “Consumption” was the leading cause of death at the time. Depending on the decade and the nation, it was responsible for one in every eight deaths, one in six, sometimes one in three. The only consolation, if you could call it that, was that the damage took hold and progressed fairly slowly, allowing people to remain functional for up to five years or so.
But what caused it? Nobody who became afflicted with the disease ever had any idea where or when or how their affliction began. (As we now know, symptoms can appear months or years after infection.) Was consumption due to some flaw in nutrition? Was it an inherited disease? Nobody knew for sure.
Sometimes doctors would examine the lungs of people killed by consumption, and they would find small lumps or “tubercles”. In 1834, the disease began to be known by a new name: tuberculosis. In 1869 a French scientist caused tuberculosis to appear in rabbits by injecting them with matter taken from diseased humans or cattle, thus showing for the first time that tuberculosis is actually transmissible and contagious. If anthrax is a contagion caused by a living parasitic microbe spreading from host to host and infecting one after another … maybe tuberculosis is, too? Robert Koch marshaled his newly invented methods, techniques, and skills, and redirected them at this devastation upon mankind.
Tuberculosis
Again we begin. If the disease was caused by a germ, then one should be able to find that germ in every diseased animal, but one should never find it in a healthy animal. Koch managed to get hold of a recent corpse, a man killed by a severe attack of tuberculosis, so he could search for a culprit microbe. But this time, there was no germ to be found. The anthrax bacilli had been fairly easy to stumble across. These tuberculosis germs, if they were there at all, were well hidden.
Anyone who has ever played with a hobby microscope and searched for critters in pond-water knows that the world in the window is often not very colorful. Sometimes all you see is a glowing pool of light, with faint shimmery edges of shadows here and there. It can be like looking at a clear glass bead underwater. Watery things swimming in water are much easier to see if you can paint them somehow. I’m not sure if Koch can be said to have invented the art of microscopic staining, but he was certainly one of the pioneers. In the previous few years, he had taught himself how to stain a variety of microbes with a variety of dyes. In the search for the culprit of consumption, Koch tried every stain and dye and combination he could … and eventually found a dye that revealed a new germ. Finally he found a strange, artificially-colored trespasser in the lungs of the dead man. This time, instead of thick rods and chains of rods that look like freight-trains, he found wispy thin threads, tinted faintly blue by his dye.
Now for lab work. Could he give guinea pigs tuberculosis? He tried injecting them with ground-up tubercles. They died. Had they died of tuberculosis? Guinea pig postmortems revealed new tubercles inside the corpses. Had the threads from the tubercles reproduced and filled the new victims? The microscope revealed new generations of threads thriving in the deceased guinea pigs.
Koch was nothing if not thorough. He begged diseased tissue from hospitals and morgues across Berlin, and then injected it into hundreds more guinea pigs, as well as mice, rats, rabbits, cats, dogs, chickens, pigeons, and a pair of groundhogs. He never found the wispy threads in healthy animals, only in animals that died.
Koch had his suspect. He had a candidate for the germ of tuberculosis. As before, now Koch needed to isolate, purify, and culture this suspect microbe, and then inoculate an animal with pure-bred cultures, to see if this created tuberculosis in the animal. But again, this germ proved more truculent than the anthrax bacilli. This one did not like aqueous humor, nor would it grow in any other culture media that Koch tried. Maybe these fussy eaters needed live food? Or at least fresh blood? Koch figured out a way to make jelly out of sterile blood serum, and he tried growing his suspected thread-germs on that. At first it looked disappointingly like another failure. But maybe it was just an unusually slow-grower? After all, tuberculosis can take years to kill a person. Koch decided to wait and see. After two weeks, he finally saw spots. Tiny colonies of something had grown on the blood-jelly. Were these spots little cities of the suspect threads … or were they something else? The microscope showed that they contained the wispy blue-tinted threads. Koch had figured out how to care for his second microbe. He could grow this one in test tubes on blood-serum-jelly. His microbe husbandry expertise had grown to two species.
Having found a suitable home for them (with microbes, food and home are much the same thing), Koch set out to isolate, to purify, to prepare that which does not occur in nature — cultures of one and only one microbe. He collected samples from dozens of his deceased animals and set about growing crop after crop, generation after generation, each time starting one culture from an extract of the previous one. After months of purification, he was ready to try the crucial test: Would an inoculation of this pure-bred microbe result in the appearance of tuberculosis? As before, one animal was not enough. In another attack of thoroughness, Koch injected his purified microbe-threads into guinea pigs, rabbits, hens, rats, mice, and monkeys, as well as animals not known to suffer from tuberculosis naturally: tortoises, sparrows, five frogs, three eels, and a goldfish. The latter group completely failed to get sick. They also refused to allow the wispy threads to fill their blood. But the guinea pigs succumbed. The guinea pigs had contracted tuberculosis, and the only change that had preceded it was the entry into their bodies of one kind, and only one kind, of microbe. Koch had satisfied another of his requirements for proof. The final requirement quickly followed. The microscope revealed the next generation of germs had filled the bodies of the newly diseased rodents. The disease and death of the guinea pigs had been accompanied by the growth and reproduction of the living germs in their bodies.
Koch was now certain that these wispy threads were the true cause of tuberculosis. But he decided to see if he could fill in one more piece of the puzzle before presenting his final case to the jury of the world’s doctors and scientists. As with anthrax, he wanted to understand the means of transmission. All of his laboratory animals had been given tuberculosis by injection, but tuberculosis is primarily a disease of the lungs. People probably caught the disease in reality, outside the laboratory, by inhaling it. Could Koch give his animals tuberculosis, not with a needle, but through the air? But … how in the world can you spray tuberculosis germs into the air safely? Koch’s inventiveness again solved the problem. He built his animals an air-tight kennel with a spray nozzle … and then succeeded in killing them with nothing but airborne wispy threads.
In 1882, Koch traveled to Berlin, and addressed a meeting of the biggest names in German science. The next day, headlines around the world trumpeted the news. The greatest killer of humanity had been found. Doctors flocked to Koch to learn how to culture the germ, how to make it appear under the microscope, how to inoculate animals with it. As with anthrax, there was still no cure, but at least the dread disease could now be effectively diagnosed, and intelligent measures could be taken to avoid it and keep it from spreading.
Robert Koch had caught the culprit of consumption — the germ now known as Mycobacterium tuberculosis. He had proven a cause and effect relationship a second time. He had now logically, persuasively, and permanently identified the microbial cause of two major diseases.
Cholera
In the 1800s, epidemic diarrhea or “asiatic cholera” simmered quietly in India, with occasional sorties into neighboring lands. In 1883, outbreaks erupted in Egypt and threatened Europe. Pasteur by this time had been spurred into action by Koch’s discovery of the anthrax bacillus and had shifted his full time energies into discovering and destroying the causes of disease. When cholera erupted in Alexandria, both Germany and France send a germ-hunting team to Egypt to try to find the cause. Pasteur sent a couple of his assistants, and Robert Koch went himself, with one of his own assistants. It was an odd sort of competition, inflamed by Franco-Prussian rivalry, but also strengthened I think by respect between two giants fighting ultimately for the same thing. When one of the French team — a youthful newcomer named Thullier — succumbed to the deadly disease he had been studying, the German team paid their respects at the funeral. Robert Koch helped to carry the casket.
Eventually, Koch and his dyes revealed a wiggly, comma-shaped microbe, a vibrating Vibrio, in the cholera patients. With a suspect identified, Koch then traveled to India, where the disease was abundant, to attempt to prove that this was truly the germ of cholera. (Animals do not suffer from cholera, and if you can’t work with lab animals, the only thing you can do is to go somewhere where there are many sick people.)
Koch was by now an old hand at being a germ detective, and the wiggly cholera vibrio offered few of the challenges of the spore-forming anthrax germ, or the hard-to-find and hard-to-grow tuberculosis germ. Koch marched through his steps of proof fairly quickly this time. He searched every victim he could find, as well as hundreds of healthy people. He always found the vibrio in diseased patients, and never in healthy people, nor in any of the animals he searched, from mice to elephants. The germ was easy to grow and purify on standard beef-broth jelly. The only weakness this time was that Koch could not demonstrate that injection of pure cultures causes the disease, because he could not deliberately inject people.
Further investigations showed that the cholera vibrio is easily killed by drying out. Unlike the anthrax bacillus, it does not have the ability to weather bad times outside a body by transforming itself into temporary weather-proofed spores. However, unlike many parasites, it does not absolutely require a host. It can live just fine in water outside a body … as long as it can find water that sufficiently resembles the contents of a person’s intestines. Koch showed that the water tanks in many huts were harboring the germ, and that it could sometimes be transferred by soiled linen. This ability to live in foul water meant that the parasite didn’t have to be too aggressive about finding a new host right away. But it also meant that cholera, unlike the spore-forming anthrax or the airborne tuberculosis, could be easily roadblocked by basic sanitation. Modern water and sewage treatment systems have virtually exterminated cholera in developed countries.
For a third time, with rigor and thoroughness, with “order and method”, Koch had proven a cause-and-effect relationship — this time between the disease of cholera, and the bacteria we now call Vibrio cholerae.
Koch’s Rules of Proof
Louis Pasteur was inspired (or perhaps provoked) by Koch’s discoveries to return his attentions to the war against human diseases, and Pasteur would go on become a giant in the business of combating disease and a savior of perhaps millions of lives. But Koch was King when it came to rigorous proof. Koch’s conviction of those first three germs of disease wiped away confusion and debate, and allowed future disease-fighters to concentrate their fire where it belonged — on the germs that cause the disease.
But constructing certainty is like constructing a skyscraper. It is lengthy and difficult. Consider how careful and methodical Koch had to be to prove his case: He always started by identifying a suspect germ, an unusual microbe in a diseased patient. He then showed that this suspect microbe is always present in diseased animals, and never in healthy animals, in as many examples across as wide a range of conditions as possible. He then found a way to isolate and purify this microbe, and grow purebred crops of it on some kind of organic food in a test tube. This confirms that it is a living thing, and enables the following step. Perhaps the most convincing step in the proof is to prepare a pure injection of that microbe, and only that particular microbe, and show that the inoculation is followed by the appearance of the disease. The effect follows the cause. If the only thing that changed was the introduction of a particular microbe, one can be pretty certain that that microbe was the physical cause of the subsequent disease. Finally, Koch would re-isolate and re-identify the microbe from the blood or the tissues of the sickened animal. This shows that the microbe has reproduced, and filled the body as the body got sick. Koch didn’t yet know how microbe anatomy worked, or the precise way in which the germs caused the damage, but at least he could show that their reproduction corresponded to the progression of the disease.
Koch always followed this methodical, rigorous procedure, and he always ended with conviction. If you want your conclusions to be sound, if you want your quest for knowledge to end in certainty, if you want your actions and choices to be founded on solid ground … this is what you must do.
Later in life, Koch settled into government advisory work, and published his rules of germ-hunting in a formal way. These rules of proof became known as “Koch’s postulates”. As Galileo may have inherited some ideas from Renaissance thinkers before him, perhaps Koch inherited some ideas from Francis Bacon or John Stuart Mill. Koch’s Postulates certainly bear a resemblance to “Mill’s Methods” for inducing a cause-and-effect relationship. But like Galileo, Koch built his own legacy. He was responsible for the triumph of the germ theory. He was the first to prove a cause-and-effect relationship between specific microbes and specific diseases. And he did it as it had to be done — with “order and method”.
THE LEGACY OF THE GERM-HUNTERS
Galileo was an intellectual fountainhead in 17th century Italy, pouring out a river of inspiration and creativity that ran in streams through all of subsequent history. In the field of disease, I think it can be said that Pasteur and Koch were both similar fountainheads, in 19th century France and Germany, respectively. They both built world-famous Institutes and trained a generation of disease-fighters, many of whom would go on to make significant discoveries in their own right.
Pasteur had no training as a doctor, and wisely hired two medical assistants to help him through most of his disease research: Charles Chamberland and Pierre Paul Émile von Roux. Under Pasteur’s guidance, these three discovered that weakened germs can induce immunity without causing disease, and they built on that discovery to engineer the first three artificial vaccines: those for anthrax, chicken cholera, and rabies. The number of lives saved by these vaccines, and by all of the subsequent vaccines patterned after them, cannot be counted.
In 1884, Chamberland invented a new kind of water filter, with pores so small it could remove all microbes. One could examine water from this filter with the highest-power microscopes and find no life. It was intended to supply the lab with pure sterile water, but eventually people started using it to filter other things — germ cultures, blood serum, and such. In the 1890s, people were searching for the unidentified germs of new diseases, and they discovered that with some diseases, there was an invisible germ. You could filter the extracts from diseased plants or animals and confirm with the microscope that they contained no microbes, and yet the clean fluid still made healthy animals sick! Was there a poison instead of a germ? No. Whatever it was, it could kill animals with undiminished strength generation after generation, so it must reproduce and replenish itself in each new animal. It must be something living. Was it some kind of living poisonous fluid? Or was it a germ, but a germ so small that it could pass through the filter and couldn’t be seen even under the microscope, a germ far smaller than any known bacteria? Whatever it was, it became known as a filterable virus — using “virus” in the original sense of “unhealthy filth or slime; poison”. Later the word “filterable” was dropped, and the name “virus” was officially applied to this entirely new category of invisible germ. Chamberland’s filter had enabled the discovery of viruses, and figuring out exactly what viruses are would occupy much of the 20th century.
In 1888, Roux tried filtering cultures of diphtheria, removing the bacteria that were known to cause the disease. He discovered that the filtered culture still made animals sick, even without the bacteria! But this time, the sick animal could not infect any other animals, and no diphtheria bacteria filled the animal’s body. The animal had been harmed, not by the bacteria directly, but by the toxin they had manufactured. Before Roux, nobody knew for sure how the lives and habits of germs harmed your inner functions and made you sick. Roux showed that in at least some cases, they do so by making poison. The germs don’t attack or eat our tissues directly, they poison us with their products. As fermentation bacteria make alcohol or lactic acid, so other bacteria make lethal toxins.
Robert Koch also had colleagues, trainees, and disciples. One of the most famous of these was Paul Ehrlich. Ehrlich was a wizard with dyes. He figured out how to stain various things inside a living body, and he discovered for the first time that there are different kinds of white blood cells. He was also fascinated with the problem of how to kill a living parasite without harming the host. How do you create a drug that will attack only the things at which it is aimed, and nothing else? Ehrlich searched far and wide for these “magic bullets”, and eventually created the first antibiotic drug — a syphilis treatment called Salvarsan.
As the 20th century dawned, so did the age of modern medicine. Many diseases caused by the microbial “whales”, the protozoa, would soon be wiped out by sanitation. Other protozoal diseases, like malaria and sleeping sickness, would be stopped by pest control. (Near the turn of the 20th century, it was discovered that many germs, especially protozoa, hitch a ride from host to host in mosquitoes or flies.) To fight bacterial infections, we now have a spectrum of antibiotics … although overuse has been producing more and more resistant strains of bacteria. Broken limbs can be healed and women can give birth in modern hospitals with negligible risk of death from smelly hospital diseases.
As the 20th century dawned, however, a few perplexing puzzles remained. For one thing, not all germs were easy to find. Some were proving elusive, especially those invisible “viruses”. For another thing, we had discovered that germs cause disease, but we had also discovered that microbes aren’t rare attackers, scattered and hidden in nature. Microbes are everywhere. Instead of asking why we get sick, the new question was: why don’t we get sick? If we live our lives in an ocean of germs, why aren’t we sick all the time? What keeps us immune to most of their effects? The solutions to these two puzzles would make fascinating stories of their own …
While doing my own research in preparation for writing this article, I gathered information and took notes from many sources. But the one source that I relied upon most was the classic book Microbe Hunters, by Paul de Kruif. If you have read it, you will recognize my debt to it. If you haven’t, I recommend it. (Another recommended book — very brief and suitable for younger readers — would be How We Found Out About Germs, by Isaac Asimov.) The following quote is de Kruif’s description of what Robert Koch had accomplished with his anthrax lecture in 1876. This is what Koch and all of his predecessors managed to achieve in their quest to conquer disease:
“So it was that in these three days at Breslau this Koch put a sword Excalibur into the hands of men, with which to begin the fight against their enemies the microbes, their fight against lurking death; so it was that he began to change the whole business of doctors from a foolish hocus-pocus with pills and leeches into an intelligent fight where science instead of superstition was the weapon.”
This is what careful observation, relentless testing, and thorough thinking can do.
About the Creator
Enjoyed the story? Support the Creator.
Subscribe for free to receive all their stories in your feed. You could also pledge your support or give them a one-off tip, letting them know you appreciate their work.
Comments
There are no comments for this story
Be the first to respond and start the conversation.