Unification Theory?
The underlying force behind light, gravity, and the life-cycles of celestial bodies.
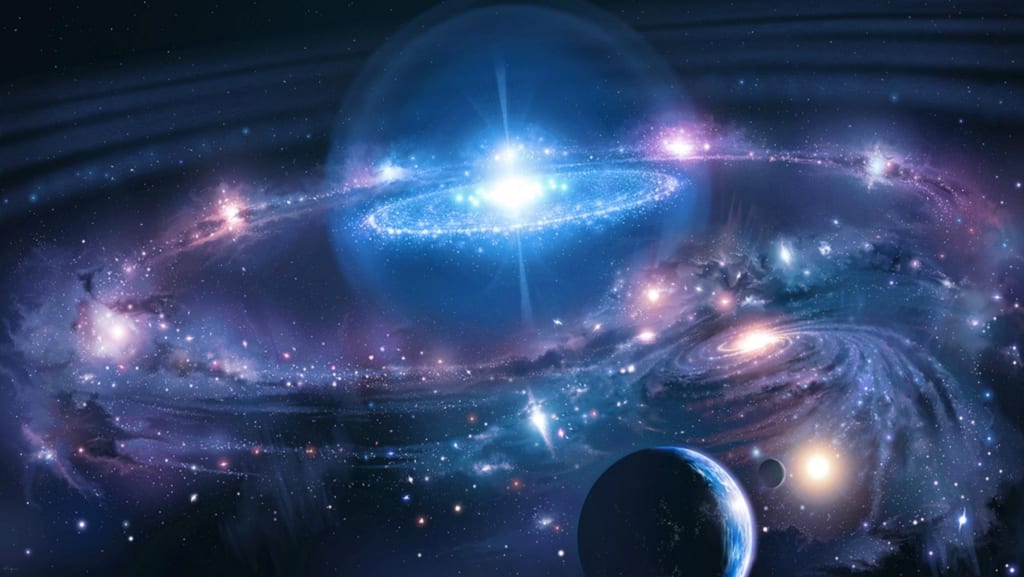
THE UNDERLYING FORCE BEHIND LIGHT, GRAVITY AND THE LIFE-CYCLES OF CELESTIAL BODIES
Pardon the overly simplistic descriptions presented here. There is a great deal of material to cover, which forces me to keep it simple and move quickly.
My objective is to present a general overview that there may be a single common force behind light, gravity and the life-cycles of celestial bodies. But please wait till the end before deliberating, as key points keep coming to the finish.
If anyone can compose a more scientific version of my theories, by all means, feel free to do with this article all that you can. I am putting it out there not to close the issue around a fixed conclusion, but to open the issue to further debate; not to close minds around one explanation, but to open minds to further theorizing.
This article progressively leads to gravity, planetary bubbles, black holes, and finally to “big” and “little” bangs. So please bear with me through this early stage and you just may be intrigued.
Seeing Light
• We begin by looking at light. It is not important to identify what light is, which relieves me of a monumental task I am unable to undertake.
• Our eyes see only one thing… light. When we look at an object, we are not seeing that object. What we are seeing is light from the nearest light source reflected off that object. As the light bounces off, its properties are altered by the various properties of the object, such as texture, colour, glossiness of the finish, moisture, heat, etc.
• As an example: imagine three rooms - one painted white, another black, another red. Each room has a single chair at its center, identical to the others. Each room also has a single white light bulb hanging from the ceiling. If the light bulbs are off, and there is no other light source entering the rooms, you cannot see the chairs. Again, we do not see objects; we see only light.
• Turning on all three light bulbs, light can now bounce off the chairs creating images of the chairs in our eyes. But the light coming to us from each chair is slightly different. The chair in the white room appears brighter, the chair in the black room appears darker, while the chair in the red room appears pinkish. While some of the light entering our eyes has reflected off the chairs directly, other light has bounced off the walls first and then the chairs before entering our eyes. With each bounce the light is changed in a small way; the light bouncing off the black walls darkens, so that when bouncing off its chair, the chair appears darker, while the light bouncing off the red walls pinkens, so that when bouncing off its chair, the chair appears pinkish. Obviously the chairs’ properties have not changed, but the light’s properties have.
• Our eyes function much like night-vision goggles. Night-vision goggles detect heat, creating images based on heat distribution. In similar fashion, our eyes detect radiation, specifically the narrow band of radiation which is to us visible light. That is all our eyes can see… radiation; we see nothing else. But our eyes are so finely tuned to radiation that the slightest change in its pattern caused by striking an object is easily detected, such as the difference between a chair’s soft cloth cushion and hard wooden back.
Light’s Path
• This takes us to the line-of-sight of light. When we look at an object, we see more than just the light striking it from a nearby light source, but are actually looking at the light source itself. Back in the rooms mentioned above, if you held a mirror under one of the light bulbs and angled it properly, you would see the light bulb when looking at the mirror. Similarly, when looking at the chair, you are seeing the light bulb when looking at the chair. The only difference is that the mirror reflects an extremely sharp image of the bulb, while the chair - because of its less reflective materials - reflects back an undefined image of the bulb as just a smear of light.
• Now imagine yourself standing under a tree by the water on a clear night with a full moon. When you look at a leaf on the tree, what are you seeing? The Sun. In the absence of any artificial light, the light reaching your eye can be traced from the leaf, to the water, to the full moon, to the Sun on the other side of the Earth. Along its path, the light from the Sun has been altered by the moon, the water and the leaf to produce a darkish silvery green colour. If that pathway of light from the Sun to the leaf is broken at any point, say a very large truck parks next to the tree preventing the leaf from receiving any light, you no longer see the leaf.
• Thus, our eyes see light only when there is an unbroken pathway from our eyes to the light’s source. Regardless of how many bounces that beam of light takes, we see nothing if we don’t have a solid unbroken line from our eye to the light source. This tells us that light can be seen only along its beam; light cannot be seen sideways.
• What do we mean by sideways? You may have seen one of those lasers that shoots light beams from one end of the apparatus to the other. Since the laser beam does not enter our eye we can’t see it. The only way to see the beam is by blowing smoke into it. And even then, the only portion of the laser beam we see is just that segment which is in the small cloud of smoke; the remainder of the beam outside the smoke is still not visible.
• The laser beam passing through the smoke reflects off the smoke particles, bouncing off in all directions. We see only those reflections that bounce in the direction of our eyes. If two people were standing with their heads next to each other, both would be able to see the laser beam in the cloud of smoke, but each of their four eyes would be receiving different beams.
• Now although it appears we are seeing the beam move sideways through the smoke, what we are seeing is in fact beams that have broken off the path of the main beam and have reflected into our eyes. We are still seeing light just along its pathway, along its beam. We are not seeing light sideways, as the segments of the laser outside the cloud are still invisible. Likening a beam of light to a drinking straw, we see light only when we are looking through the inside of the straw; the straw would be invisible if we looked at its side.
• This tells us something about the transmission of light through space… it cannot be carried by spherical balls that emit light in all directions as they move. If it were, space would be much brighter than we see it, for there are beams of light shining every which way, and we would see them all even if they did not enter our eyes. A star would not be a point of light, but would be a round circle of light getting progressively dimmer as the light moves away from its center. In fact, the whole night sky would be full of overlapping circles of light of varying sizes depending on each star’s size and distance. But as it is, we see light only along its beam, not sideways. Since stars are so very far away, only a few of their “straws” are pointing at the Earth. They thus appear as little points of light. (This may help address Olber’s Paradox, though not entirely.)
• As a side-point: Seeing as we cannot see light sideways but can see it only along its beam, might light be one-dimensional? We know that a three-dimensional object can be seen along its three planes, a two-dimensional object along its two planes, and a one-dimensional object along its one plane. Since we see light only along one plane, might that make it one-dimensional?
• As another side-point: A simple test with a vacuum tells us that light does not move as sound does... sound does not travel through a vacuum, while light does. The reason, of course, is that sound requires particles, such as atoms and molecules, to carry the wave along as they bump into one another. But in a vacuum there are no particles to carry the sound out the other side. Light, however, does pass through a vacuum tube - glass and all - and comes out the other side. Thus, it must be moving through the spaces between atoms, even the spaces within an atom between its nucleus and electrons. It must be moving through the “fabric” of space itself.
Two Significant Properties of Space
• In the simplest of terms, the fabric of space is the “grid” or “framework” or “background” of the universe. Imagine space as a gigantic mass of jello; atoms and molecules are small bits of fruit within the jello, while the fabric of space is the jello itself that weaves in and around the fruit. This space fabric fills all gaps, including the spaces between atoms, and even the space inside an atom between its nucleus and its electrons. It is through this space that light and radiation move.
• Does, now, the fabric of space react in some way to the presence of this energy within it? Or does the grid of space retain its rigidity, consistency and uniformity regardless of the distribution of energy? To answer this we need to turn to another form of energy besides light… matter.
• As the most famous formula E=MC2 tells us, matter releases light and other forms of energy. Matter, then, is energy in concentrated form. We might say that just as there are three states of matter - solid, liquid and gas - there are two states of energy - solid energy (matter) and liquid energy (light and other radiation). It is commonly accepted that large concentrations of matter, such as stars and other celestial bodies, cause space to bend, warp or fold, a fundamental concept behind gravity. It is in this way, then, that the fabric of space reacts to the presence of energy (whether solid energy in the form of matter, or liquid energy in the form of radiation) by changing shape in one way or another. Space, then, is not rigid, but has elasticity.
• This, now, adds a second property to the fabric of space… if space has elasticity, it must also have density. It is clear that the gravitational effect of a celestial body described above has limited reach; at some point in space, the Sun’s gravitational pull stops pulling things in. So the warpage caused by a celestial body will bend space out to a certain distance, leaving the space beyond that limit unchanged. This by necessity requires compression in one area of space and extension in another. Now if an area of space has had its fabric compressed, then this area of space must have a greater density than it had before. And if another area has had its fabric stretched, its density must be lesser than it was before. Density is inextricably coupled with elasticity, and these form two very consequential properties of space. So consequential, in fact, that everything we see happening in the universe happens because of them.
Gravity – Pull or Push?
• It is these two properties of the fabric of space – elasticity and density – that better explain gravity. Why “better” explain? Because the current concept of gravity falls short. Just having curves in space is not enough. Indeed, the curve steers a body as it moves; but there needs to be something that makes the body move within that curve in the first place. Curvatures in space provide the direction, but not the motion. Neither is it enough to say that matter attracts other matter, for attraction and gravity are really the same thing. It’s like trying to explain why humans can speak by saying it’s because we can talk.
• But if we see space as having more than just varying shape but also varying density - now the force of gravity has a reason, the attraction of bodies has a cause. Let us consider the “bowling ball on a mattress” illustration, which is a two-dimensional representation of gravity. As the bowling ball warps the surface of the mattress, so too a celestial body like the Sun warps the space around it. If you now place a golf ball on the mattress, and if it is close enough to the bowling ball, the golf ball will begin rolling toward the bowling ball, stopping at its side. The golf ball has taken the path of least resistance. But what was it that started the golf ball moving? What gave it motion? It was the imbalance in the level of the mattress.
• Now of course, this two-dimensional representation of gravity has a shortcoming in that the model uses the real Earth’s gravity to start the golf ball moving. The bowling ball in the model curved the mattress, but it was the real Earth outside the model that made the golf ball move. So this 2D model is still incomplete; it still does not account for that one thing that gives motion. However, it gives us a 2D shadow of the actual property that does provide motion… the imbalance. Where the 2D model had an imbalance in the mattress’ surface, the real 3D universe has imbalances in its fabric’s density.
• The part of the mattress that is higher would correspond to an area of space that has greater density, while the part of the mattress that is lower would correspond to an area of space that has less density. In regions of increased space density, the fabric of space is compressed. Compressed space, like a compressed spring, is constantly trying to return to its natural tensionless state, thus pressing down on any body within it. This exerts pressure on the body, which will begin to move if there is an area of less dense space nearby that it can move into. It therefore takes the path of least resistance, out of denser space into less dense space.
• Gravity, then, isn’t so much a pull as it is a push or a squeeze. It’s not that a large body pulls a small object toward it; it’s more that compressed space fabric pushes the small object out of that region of space fabric. And where does the object go? Toward the larger body which has already created an area of less dense space around it.
Bubbles
• But how does a celestial body cause the space around it to bend, warp, and become less dense? Let us return to our mass of jello to illustrate. Imagine each bit of fruit in the jello emitting a little heat. The heat would warm the jello around the fruit, softening it. Larger concentrations of fruit would emit even more heat, warming a larger area of jello, even liquefying it. In any given area of jello, the more fruit there is, the more heat there is, and the more liquid and less dense the jello there is. Any free moving bit of fruit would have an easier time passing through the liquidy areas where there already is a lot of fruit, than it would through the more solid areas where there is less fruit, than it would through the really solid areas of jello where there is no fruit at all. The jello, then, is of varying density throughout, depending on the distribution of the fruit within it.
• Each bit of fruit or cluster of bits of fruit has a little bubble of warmer, softer, less dense jello around it, which gets progressively cooler, firmer and more dense as it extends outward away from the fruit. It is as though the fruit were pushing the jello away from it, clearing room around it and gaining greater freedom of movement within its little area of less dense jello. Looking at the jello mass from afar, you would see little “jello bubbles” around each bit or cluster of fruit, and the more bits of fruit there are massed together, the larger their bubble would be.
• All celestial bodies bend and warp the area around them simply by occupying space, while some bodies such as stars extend their area of influence further by emitting radiation outward. Whether by solid energy alone (just with their atoms) or by a combination of both solid and liquid energy (their atoms and their radiation), all celestial bodies “warm” the area of space around them, thinning it out and lessening its density.
• The question now is… why? What is the cause of the thinning of space and the formation of these bubbles of lesser density? The only cause I can come up with is the vibration of atoms. As atoms vibrate against the fabric of space, they rub off some of their energy into that fabric; let’s call it sub-atomic friction. This may account for atomic decay, like an eraser losing some of its mass to the sheet of paper it is rubbing against.
• By “warming” and thinning the space around them, then, celestial bodies are in a way pushing some of the fabric of space away from them, kind of like a rolling pin pushes away dough as it repeatedly rolls over an area. However, beyond the body’s area of influence, the density of space is normal. Thus, between these two regions – the point at which the celestial body’s radiation field meets the rest of unaffected space – there would form a narrow ridge or membrane of very dense space fabric that is an accumulation of the space fabric that was pushed away by the body’s energy, kind of like the snow hedges left behind by snowplows.
• The universe itself is a bubble, the greatest bubble of all. Think of the universe as a gigantic rubber balloon, while energy and matter are the air inside it. The rubber balloon is constantly trying to shrink and return to its natural tensionless state, while the air inside it is constantly applying an opposing force to keep the balloon expanded. You can never have a portion of the balloon where there is only rubber without air within it, just as you can never have a portion of the balloon where there is only air without any rubber around it. Because of this, the range of space densities within any bubble would run from >0% to <100%. You can never have 0% density, for that would require just energy without any space fabric around it. Conversely, you can never have 100% density, for that would require just space fabric without any energy within it. The density within the bubble would range from least dense at the very center of the body (thinnest and least resistant to passing objects) to most dense at the ridge where the bubble meets the rest of space (thickest and most resistant to passing objects).
Motion Through Bubbles
• Let us test drive this infrastructure we have built and fly a comet through it. The comet begins its journey as a rocky mass as far out as the Kuiper Belt, where according to the 2D mattress model the slope of space is almost completely flat, and according to the 3D jello model the density of space is just a tad below 100% and almost solid jello. At this distance, the Sun’s “gravity” – or according to our definition, the warpage of the Sun’s space fabric caused by its mass and radiation – is extremely weak. The Kuiper Belt is still within the Sun’s bubble of space fabric, but only barely; the belt is on the almost perfectly flat part of the mattress.
• As our soon to be comet revolves around the Sun in a counter clockwise direction, the density of the space on its left (toward the Sun) will be ever so slightly less than the density of the space on its right (away from the Sun); in the 2D model, then, space is tilted slightly to its left. Ever so slowly, over a period of many millennia if not millions of years, the constant squeeze applied to the rocky mass by the space fabric around it pushes it into the less dense space fabric to its left, toward the Sun, picking up speed as it ventures deeper into an ever decreasing slope in the 2D model, an ever decreasing density in the 3D model. It is now a bonafide comet on an increasingly more direct path of least resistance toward the Sun.
• As the comet rounds the Sun and starts to head back outbound, it is actually going against the laws of motion in that it is now moving into resistance, out of the less dense space fabric into increasingly more dense space fabric; it is rolling up the slope on the mattress model. This is possible due to the momentum the comet picked up during its approach to the Sun. But this momentum gradually wears off, and the comet’s speed slows as it continues moving outbound. It is not that the Sun is pulling on the comet to slow it down, but it is the increasing density of the space it is flying through that slows it down as the fabric presses down on it from all sides, much like a submarine descending into deeper water. Once the increasing density of space has slowed the comet down enough, the body will once again be squeezed into a path of least resistance toward the thinner, less dense space fabric toward the Sun.
• This leads us to the three main principles governing the motion of celestial bodies within the fabric of space… a) the body will primarily take the path of least resistance, out of greater space density into lesser space density, b) if the body has enough momentum, it can move against resistance for a time, out of lesser space density into greater space density, and c) the body’s momentum can increase or decrease according to the density of the space it is travelling through.
• As a side-point: If the density of space can alter the speed of comets and other bodies (which are solid energy), might it also alter the speed of light (liquid energy)? If space were always rigid and completely uniform, then the speed of light would be constant. But if space density varies, might the speed of light through it likewise be variable? This would make measuring the distance of stars much more complicated, and may even question the idea that everything is moving away, as the red-shift in the spectrum of light received from these bodies may not be caused by their movement, but may rather be caused by their light travelling through pockets of denser space fabric - much like the reason for reddish sunrises and sunsets. The Doppler effect observed, then, may instead be evidence of the varying density of space.
Bubbles Within Bubbles
• And so the comet, now in an elliptical orbit around the Sun, travels around our star again and again. Until one time it happens to fly a little too close to one of our larger brothers, say Jupiter. What the comet does next depends on how fast it is travelling and how deeply it flies through Jupiter’s bubble. Jupiter has a bubble too? Jupiter has a bubble too. All the planets do. The same principles causing the Sun to have a bubble are at work in all celestial bodies.
• If we wanted to represent the changing density inside one of these bubbles, we might pick a scale of >0 to <100 percent (again, the absolutes of 0 and 100 are not possible). The scale begins at slightly above 0 at the body’s core - where matter is the most dense and space fabric is the least dense - and progressively increases until it is equal to the space density outside the bubble. Let’s call this outer-most area of the bubble the “ridge”, the point at which the bubble ends.
• The comet would continue to follow the path of least resistance all along its path, out of denser space and into less dense space. It will veer off into any even less dense space it encounters; thus, the path of least resistance is altered by the movement of smaller bubbles within larger ones. Now if the comet has enough momentum, it would fly completely through Jupiter’s bubble of less dense space and come out the other side, with its trajectory altered only slightly. This happens to artificial satellites and probes that are intentionally flown close to a planet to pick up speed and change trajectory. However, if the comet or probe is not moving quickly enough to clear the smaller bubble, its trajectory will curve sharply inward toward the planet and keep the object forever trapped inside that planet’s bubble, perhaps even drawing it into the planet itself.
Density Equilibrium
• When we look at the planets of our solar system through a telescope, then, we might imagine little bubbles of thin, liquidy space all around them, much like the bubbles of liquidy jello around those clusters of fruit bits in the 3D model. The size of these planetary bubbles is directly proportional to their planets’ mass; the more mass the planet has, the more space fabric it heats up, thins out and pushes out, extending the reach of its bubble. Meanwhile, the Sun’s own space bubble envelops them all; planetary bubbles within an all-enveloping solar system bubble.
• But planetary bubbles within our solar system can explain more than just how planets capture objects like comets and moons; they may also account for how the planets are spaced from Mercury to Neptune. It all rests on “density equilibrium”. Here, we’ll need to recruit the aid of two examples… First, an empty plastic bottle containing nothing but air. If the air were sucked out of the bottle, the air pressure inside the bottle would be less than the air pressure outside the bottle, and the bottle would crush. Once the air is allowed back in, the pressure inside and outside the bottle equilibrate, and the bottle resumes its normal shape. Second, two large soap bubbles blown into the air that have joined together. The bubbles enter into each other to an extent, much like the two circles on the MasterCard credit card. But between the two bubbles there is a flat membrane dividing them and keeping their inner chambers separate.
• Similarly, if two planetary bubbles touched each other, both would crush into each other just a little. The bubbles would stop moving into each other at the point where their densities are equal, say 50-50, or 40-40, or 60-60, depending on how much force is pushing the two bubbles together. If the surrounding space fabric force pushing the two bubbles together is weak, they may stop penetrating each other’s bubbles at 80-80, or 70-70 density. But if the surrounding space fabric force is strong, they may continue penetrating deeper into each other’s bubbles up to as much as 20-20 or 10-10 density. If one of the bodies is revolving around the other, their bubbles would be preventing them from colliding, as might be the case with the Earth and Moon.
• The principle of density equilibrium between two or more bubbles could be what is keeping the planets of our solar system in their orbits, and at their spacing. Imagine a cross-section of the solar system, with the density of the Sun’s bubble of space fabric ranging from 1% dense at the Sun’s core to 99% dense at the Kuiper Belt (ignoring the density of space outside the Sun’s bubble where it rests within the Milky Way’s bubble; yes, galaxies have their own bubbles too). Now the distribution of the Sun’s space fabric density is not evenly and progressively spaced, just as the warpage of the mattress caused by the bowling ball is not regular, but curves ever more steeply toward the ball. Thus, the density of the Sun’s space fabric bubble would grow from 1% to 10% very quickly, then take longer and longer to reach each 10% marker after that. Around each planet too there is a bubble of space fabric ranging from 1% density at its core to 99% density at its ridge on a similar curving-scale, the size of which is proportional to each planet’s mass.
• For ease of explanation, let’s say each of the Sun’s eight planets is positioned at a multiple of 10% space fabric density... Mercury at 10% density, Venus at 20% density, Earth at 30% density, etc. In keeping with the principle of density equilibrium between two bubbles, each planet’s orbit would be held in place as the planet’s bubble maintains density equilibrium with the density of the Sun’s space fabric around the planet. This would explain why each planet in succession is farther away than the previous – they have larger bubbles and thus command a larger area within their bubble, each bubble stopping at the point where its density matches the density of the space around it.
• So if Mercury is on the solar system’s 10% density ring, Mercury’s bubble would be extremely small, extending from 1% at its core to 10% density where it matches the density in its immediate vicinity; there its bubble stops and there the planet’s orbit is held in place close to the Sun. If Venus is on the solar system’s 20% density ring, Venus’ bubble would be a little larger, extending from 1% at its core to 20% density where it matches the density in its immediate vicinity; there its bubble stops and there the planet’s orbit is held in place at a farther interval than Mercury. And so on until reaching Neptune, which has the largest bubble of all our planets. If Neptune is on the solar system’s 80% density ring, Neptune’s bubble would extend from 1% at its core to 80% density where it matches the density in its immediate vicinity; there its bubble stops and there the planet’s orbit is held in place with the greatest distance between it and its last inward neighbour. This may also help explain why the inner planets have fewer moons while the outer planets have more… because the outer planets have larger bubbles of space fabric, covering a wider area, enabling them to trap and hold more satellites.
• The solar system’s density distribution might also explain planet size and density. A body’s material density is inverse to its space fabric density; that is, at a planet’s core its matter is most dense, making the fabric there least dense, while at the planet’s surface its matter is less dense, making the fabric there more dense. This creates a tightly packed core at the center of a celestial body, with gradually decreasing density of matter toward its surface (ie: iron at the Earth’s core, water at its surface, and air above its surface). The heavier elements keep sinking toward the core because the energy down there has thinned-out space fabric the most, making it the easiest region for matter to travel through, with heavier elements pushing lighter elements out of their way.
• Given this inverse relationship between material and space fabric densities… since Mercury is sitting at the Sun’s 10% density ring, the fabric there is thinner and less dense, allowing Mercury’s atoms to move in and pack more tightly together; whereas Neptune at the Sun’s 80% density ring has some very thick space fabric getting in the way of its atoms, preventing them from compacting as much as on Mercury. As a result, we have rocky planets at the inner solar system and gas giants at the outer solar system - all because of the outlying density of the fabric each planet is in. Think of warm and cold air masses on Earth. Warmer air is thinner and more expanded, and thus can contain more moisture; while cooler air is thicker and more dense, and thus cannot hold much moisture. The inner planets are in “warmer” and less dense space fabric, while the outer planets are in “cooler” and more dense space fabric. They can all have solid iron cores, because the center of a planet is always extremely “liquid” and minimally dense. But the farther away a planet is from the Sun, the thicker the fabric density in its vicinity is, and the more quickly the planet will lose density from its core to its surface. Thus, its outer shell extends farther, for the thicker space density around it cannot contain as much matter or energy. In this case, the planet’s material needs more space, and is thus larger and less dense.
• As a side point: The existence in our solar system of both fully formed bodies (planets and moons) as well as debris fields (asteroid belt and planetary rings) tells us that at some point in the solar system’s history there was a dividing line before which “clumping” occurred and after which clumping no longer occurs. Clumping continued throughout the solar system while the space within it was “warm” enough for rock to fuse together, early in the system’s formation, when there was still a lot of dust and gas within its cloud. All of this energy evenly spread throughout the cloud would have thinned-out the fabric of space everywhere, making it easier for atoms to sink deeper into other bodies and clump together. But as the cloud was gradually emptied of its material, the energy throughout the solar system became more concentrated within the planets and moons. This caused the density of the solar system to be less uniform and more “pot holed”, that is, where each planet and moon formed a little “pot hole” or gravity bubble, while the spaces between planets became cooler and more condensed. After this period of clumping was over, any large collisions between planets or planetoids would have created debris fields that would forever remain fragmented, never coming back together again due to “cooler” and more dense space which inhibits the clumping of rock.
Blowing and Bursting Bubbles
• The mechanism described above – where matter expands space, draws in more matter, which expands space more, which draws in more matter – can either be finite or loop perpetually. In the cases of most celestial bodies, the cycle is finite. A planet or star will grow as it draws in more dust and gas from its cloud until all of the available dust and gas in its bubble run out.
• In the case of star, the body will have grown so large, the space fabric at its core will have thinned out so much, and the atoms would have room and freedom to move so fast that any collisions would cause them to fuse together, releasing energy and igniting the body in the process. And thus a star is born. But toward the end of its life, the fusion process would have exhausted the star of its fuel, leaving very little matter behind to keep the star’s bubble extending so far out. Remember, energy is required to keep the fabric of space expanded; without as much energy left, the star’s space fabric springs back for its elasticity and begins to compress inward, like a balloon having its air let out.
• This depletion of energy at its core might release a small shockwave from the star’s center outward, possibly enlarging the star as some of its matter rides the shockwave outbound. But the star’s bubble of space fabric is still shrinking as the star simply does not have enough energy to keep it “inflated”. You now have a small shockwave moving out while the space fabric bubble is collapsing in. When the outbound shockwave finally reaches the ridge at the edge of the shrinking bubble, the two forces collide into each other head-on. You have an event similar to a baseball bat hitting a baseball… the follow-through motion of the bat (the increasing power of the collapsing space fabric bubble) would cause the ball (the decreasing outbound shockwave) to reverse direction and increase its force dramatically. You now have a huge shockwave turned around and headed back in toward the core, packing an enormous punch.
• How powerful a punch? Consider the power of a wave. In the case of light moving away from a star, we know that the light wave becomes dimmer as it moves out into space because its spherical “surface area” progressively occupies a greater area as it moves. Put more simply, if you put a 360 degree protractor on the star, the distance between any two degrees would be “y”. But at a light year away, the distance between any two degrees would be “x” times “y”. The star’s light would be weaker, dimmer, as the same amount of light is now filling a much larger area. Now imagine this process in reverse, only this time we replace the light wave moving out with a shockwave moving in. That shockwave would gain power as it moves toward the center of the star, as the same amount of shock would be concentrated over a much smaller area, moving completely through whatever matter remains at the star’s core, until the wave comes together at one singular point the size of an atom… with catastrophic effects.
• How catastrophic? First, consider a glass of water slammed on a table. A shockwave in the glass of water would move inward from the edge of the glass toward a single point at the center of the glass, ejecting some of the water clear into the air. In similar fashion, the inbound shockwave in a dying star would produce an explosion so large it would shatter the star, completely disintegrating the matter within it and sending it spraying outward. Second, consider warm air saturated with moisture. Were that air to suddenly begin cooling and contracting, the moisture within it would begin condensing and be ejected as rain. In similar fashion, the collapsing bubble of space fabric would cause the fabric within the star to condense, ejecting all of its matter outward into space. The star has gone nova. In the aftermath, the density of the space fabric that once occupied the star’s bubble returns to a cooler, thicker density, becoming more uniform as the exploded star’s matter spreads out thinly into a cloud of dust and gas.
• But what about the perpetual looping outcome? The above is what would happen to a star whose material runs out. But what if the material feeding the star does not run out? What if the star grows so large that it manages to draw in fresh material from outlying clouds, or even to steal it from the surface of other nearby stars? If a growing star has access to more material, the mechanism described above would not have to stop. Matter draws in, space expands, drawing in more matter, expanding space more, drawing in more matter… Such a star would not stop growing; the larger it gets, the farther its gravity bubble extends, putting ever more material within its reach. Frightening thought? That’s why we have come to dread this type of celestial body, ominously branding it a “Black Hole”.
• At some point throughout its life, though, even black holes may die and explode as stars do, producing a shockwave that moves out, only to be hit back in by the collapsing bubble of space fabric around it, completely shattering its nucleus and ejecting all its material into space. But what might cause a black hole’s shockwave? There could be a sharp change in the core’s “temperature” if the hole swallowed a large body or material that was “cooler”, or less energetic. This would be like dropping an ice cube into a bowl of hot soup. While the ice cube melts, it cools the soup around it. So too the slower moving atoms of the newly swallowed body or material might to a large enough extent slow down the vibrations of the warmer atoms already at the hole’s core. This change in energy output could send a shockwave outward, and the drop in energy “friction” could cause the hole’s space fabric to begin collapsing and shrinking, producing a shockwave the power of which would depend on how quickly the core’s temperature changed.
• Or, the shockwave might be caused by fission. Over time, all the material drawn into the hole might become so massive, so compacted, and displace so much space fabric, that the core’s atoms lose their cohesion and even break-apart at the sub-atomic level, leaving a void in their place, sending a shockwave out and causing the hole’s space fabric bubble to begin its collapse.
• Because a black hole requires a steady supply of material that can be siphoned off other nearby bodies or masses, a black hole would form only where there are a number of bodies in close proximity to one another, such as at the centers of galaxies, which is why many astronomers believe there are one or more black holes at every galactic center. Really, it is inevitable that there would be black holes wherever there are large concentrations of matter, for theoretically, this cycle of growth, expansion, drawing-in more, expanding more, etc, would have nothing to stop it as long as there is material available. But when that material is all used up and there is nothing left nearby, the hole will stabilize and stop growing.
• As a side-point: If the incoming shockwave rushing into a black hole’s core is not powerful enough to shatter the core, the collapsing space fabric would still be able to squeeze some of the hole’s matter outward, though in a more controlled release, similar to a volcano releasing pressure slowly through fissures rather than all at once through its top. This might explain certain phenomena such as stars with plumes of matter ejecting from their poles.
• As another side-point: If a black hole’s core’s space fabric density is so thin as to cause atoms to lose their cohesion and fragment (not enough fabric pressure to hold the particles together), perhaps light may itself fragment. This may be why black holes are, well, black. It’s not that light cannot escape a black hole; rather, light is being destroyed. Earlier we suggested that similar to there being three states of matter (solid, liquid and gas), there seems to be two states of energy (solid energy as matter, and liquid energy as light and radiation). Well, if matter can be ripped apart to produce light and radiation, perhaps light and radiation can further be ripped apart to produce something we haven’t yet discovered. Energy would, then, have a third state - let’s call it gaseous energy to fit the pattern; that is, light and radiation thinned out and broken down to an even finer state requiring such thinness of space as can be found only at the core of black holes.
Big and Little Bangs
• When black holes expire in these catastrophic explosions they would produce “little bangs” as they eject their material outward. Galaxies might be little on-going cycles of outward explosions and inward collapse, with a black hole or cluster of black holes driving it all from their centers.
• But what if there were a time when there was a dominance of black holes in the universe, each one sucking in all the available matter around it so that all the free moving matter in the universe were used up? The space fabric bubbles of the black holes would extend far enough outward to overlap one another, drawing themselves closer to one another. They begin feeding on one another, cannibalizing themselves until there remained one immensely ginormous black hole with all the matter and energy in the universe inside it, concentrated in one small sphere at the hole’s core, while all the space fabric of the universe would be thinned-out and stretched out an untold number of millions of light-years across.
• The space fabric at the core of this universal black hole would be stretched and thinned out to the point of the fabric’s maximum allowable stretch. Even so, the universe would be stable. Until one day, within one second of that day, within one googolplex’th of that second… there suddenly occurred an imbalance at the hole’s core. Perhaps every atom in the core had been broken down to its most basic sub-sub-sub-sub-sub atomic structure from which it cannot be broken down any more (which is probably pure energy in its most liquid – or gaseous - form possible). Whatever the cause, the imbalance would emit a shockwave from the hole’s core outward, creating a small void at its focus (void meaning a point of space fabric without any energy in it, which under normal circumstances is impossible, like an inflated balloon without any air in it), triggering the immediate snapping back of the universe’s space fabric.
• Already stretched to its maximum tolerance, the universe’s fabric begins collapsing inward at tremendous speed. When the outbound shockwave strikes against the incoming bubble’s ridge, you have the grand-daddy of all homerun hits. The shockwave reverses direction with tremendous velocity and power, growing ever more powerful as it rushes inward, concentrating its force into an ever smaller sphere, until it smashes together at one tiny central point at the hole’s core, producing the largest explosion the universe could ever muster… the Big Bang.
The Shape-Shifting Glove
• Although the universe immediately prior and immediately after the Big Bang may have been completely uniform and spherical in shape, there is no reason to expect it still retains that uniformity today. As explained previously, we know that matter and energy are not uniformly distributed throughout the universe, and if the arguments presented here have been effective, we might expect the universe’s density and shape to be non-uniform as well.
• Now if the stretch in the fabric of space is dependant upon the concentration of matter and energy, might the shape of the universe be irregular, such as an inflated rubber glove with fingerlike protrusions of space fabric and matter within them? And might each protrusion go through its own cycles of extension and compression, driven independently of the others by the same forces that drive matter creation (fusion) in stars and matter destruction (fission) in black holes? Some of the universe’s protrusions could be expanding and stretching, while others could be shrinking. Some might disappear all together while new ones form elsewhere as matter and energy shift and the fabric of space stretches and contracts from region to region. And at the heart of every protrusion lay a black hole or cluster of holes driving it all.
• As one final side-point: The elasticity of space may explain other phenomena which continue to confound us. For lack of a better explanation, we attribute these phenomena as the work of “dark matter”, which generally speaking provides an equal and opposite force against gravitation; dark matter pushes to counter gravity’s pull. If, however, we have the “warmth” of matter and energy “thinning” and expanding space fabric out, while the elasticity of space fabric pulls fabric back in, dark matter would not be needed, and the force we interpret as “gravity” would be reassigned work as a pusher, not a puller (as explained in its own sub-heading). At this point I wish to repeat one of the opening thoughts… If anyone can compose a more scientific version of my simplistic theories, by all means, feel free to do with this article all that you can. I am putting it out there not to close the issue to a finite conclusion, but to open the issue to further debate; not to close minds around one explanation, but to open minds to further explaining and theorizing. Let the scientific community do what it does best… absorb, revise, and re-submit for further consideration. Perhaps the ideas presented here may be useful, even if only to jar our minds to think some thoughts we never thought before.
Joseph Cafariello
Comments
Joseph Cafariello is not accepting comments at the moment
Want to show your support? Send them a one-off tip.