Unleashing the Power of Quantum
Illuminating the Future of Technology
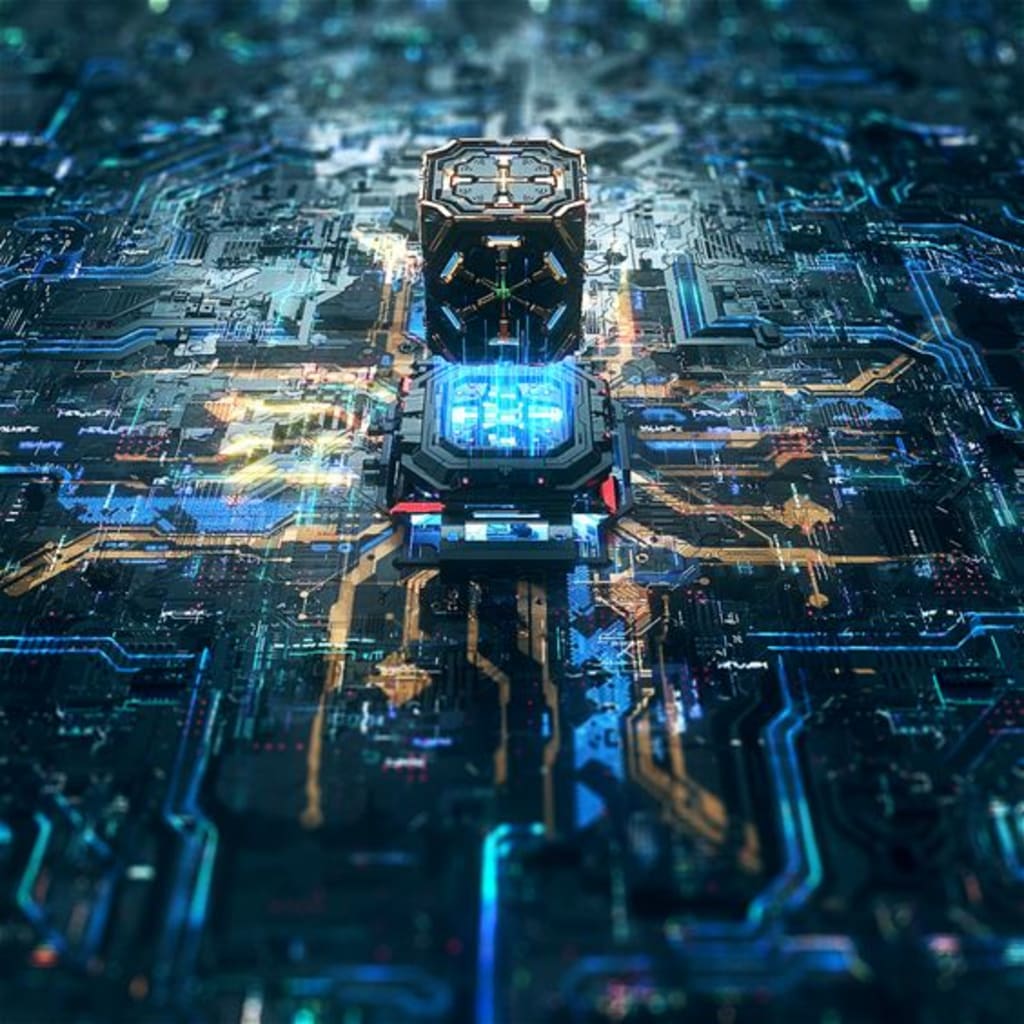
The sector of technology known as quantum computing has made significant strides and has the potential to completely alter how we handle and evaluate data. Quantum computers, which use quantum bits, or qubits, instead of the binary bits used by conventional computers, operate on the basis of quantum mechanics. This article examines the fundamental ideas underpinning quantum computing, its potential uses in a variety of fields, as well as the difficulties and opportunities that still lie ahead.
By demystifying complex ideas and offering applicable real-world comparisons, we will go deeper into the core ideas of quantum computing in this section with the goal of making them understandable to a wider audience. Superposition is one of the cornerstones of quantum computing. Quantum bits, also known as qubits, can simultaneously exist in a superposition of both states, in contrast to classical bits, which can only be in a state of 0 or 1. It's comparable to having a coin that can appear to be both heads and tails simultaneously. Qubits can represent numerous possibilities and perform concurrent computations because to this special characteristic. To comprehend superposition, consider the image of a spinning coin. The coin seems to be both heads and tails as it is spinning until it lands and unfurls into one position. Qubits can store several states concurrently up until a measurement is taken.
Another key idea in quantum computing is entanglement. When qubits are entangled, their states are organically linked to one another, regardless of how far off they are from one another. Even when they are separated by great distances, alterations made to one qubit immediately affect the state of the other. To comprehend entanglement, consider a pair of gloves as an analogy. No matter how far apart two gloves are from one another when they are entangled, you may measure the color of one glove and instantly know the color of the other glove. In a similar way, entangled qubits are intimately connected, enabling the exchange of knowledge and correlations.
In traditional computing, logic gates carry out bit operations like AND, OR, and NOT. Quantum gates are used in quantum computing to control qubits and carry out quantum operations. These gates, like the CNOT gate or the Hadamard gate, allow for actions like superposition, entanglement, and changes between various quantum states.
To comprehend quantum gates, use a toolbox as an analogy. Quantum gates act as tools for manipulating qubits, just as different tools are used for specific tasks. Complex quantum circuits can be built thanks to the individual functions of each gate. These accessible analogies and other simplifications in the text can help a wider audience understand the basic ideas behind quantum computing. It is essential to comprehend superposition, entanglement, and quantum gates in order to fully appreciate the promise of quantum computing and its capacity for parallel calculations, which will result in exponential computational power and ground-breaking applications in a variety of sectors.
Because quantum computing can solve difficult issues that are beyond the capabilities of conventional computers, it has the potential to transform scientific study. We shall examine the possible influence of quantum computing on scientific inquiry in this part, concentrating on its uses in areas like chemistry, physics, and drug development. Insights into the structures, relationships, and characteristics of molecules and atoms can be gained from quantum computer simulations of their activity. The exponential expansion in processing resources needed makes it difficult for traditional computational approaches to simulate complicated chemical systems with sufficient accuracy. The quantum character of chemical systems can, however, be handled in quantum simulations, allowing for more precise predictions of chemical reactions, catalysts, and molecular dynamics. Researchers may now better comprehend how medications interact with their targets at the molecular level thanks to the use of quantum computers, which, for instance, can model the behavior of complex compounds used in drug development. This may result in the development of more potent medications and individualized healthcare.
Even on conventional computers, modeling and understanding quantum systems is difficult by nature. However, because quantum computers may be used to control and harness quantum phenomena, they are useful resources for researching and simulating quantum systems. This has important ramifications for areas like quantum optics, quantum materials, and condensed matter physics. Researchers can explore the behavior of materials with unusual properties, such as superconductors, topological insulators, and exotic states of matter, using quantum simulations. Quantum computers can offer insights into these systems' fundamental features by simulating them accurately, potentially revealing novel materials and technologies. Extensive computer modeling and screening of enormous chemical regions are required in the process of developing novel medications or creating improved materials. By effectively exploring chemical spaces and foretelling the characteristics of molecules or materials, quantum computers can speed up this process.
Quantum computers have enhanced their capacity to predict reaction rates, optimize chemical reactions, and research potential drug candidates. They can also aid in the development of novel materials with specific properties, such as improved materials for energy storage or catalysts for ecologically friendly activities. Scientific researchers can obtain a deeper knowledge of complicated systems, speed the development of novel medications and materials, and create discoveries in a variety of scientific disciplines by exploiting the potential of quantum simulations. It is crucial to highlight, however, that quantum computing is still in its early phases, with the development of effective quantum algorithms and error correction methods ongoing. Nonetheless, the prospective influence of quantum computing on scientific research is promising, and it is driving progress in the field.
This section addresses the potential consequences for cybersecurity by focusing on the influence of quantum computing on encryption algorithms routinely employed to secure sensitive data. To provide security, traditional encryption methods such as RSA and ECC (Elliptic Curve Cryptography) rely on the difficulty of factoring huge numbers. Quantum computers, on the other hand, have the ability to crack current encryption schemes by employing Shor's algorithm, which can effectively factor enormous numbers. There is a rising need for the research and deployment of post-quantum cryptography to ensure data security in the post-quantum era. The goal of post-quantum cryptography is to provide encryption algorithms that are resistant to quantum computer assaults. These new cryptographic methods, such as lattice-based cryptography, make use of mathematical difficulties that are difficult to answer even for quantum computers.
Quantum computing has the ability to solve complicated optimization problems more quickly than traditional computers in the context of optimization. Numerous fields, such as supply chain management, route optimization, and portfolio optimization, are plagued by optimization issues. A wide number of potential outcomes can be explored simultaneously using quantum algorithms as the Quantum Approximate Optimization Algorithm (QAOA) or the Quantum Annealing, enabling quicker and more effective solutions. Quantum computing, for instance, can assist in finding the most effective asset allocation by taking into account several aspects at once in portfolio optimization, where investors seek to maximize returns while managing risks. Quantum algorithms can optimize resource allocation, save costs, and enhance logistics planning in supply chain management. Similarly, quantum computing can find the most efficient routes in route optimization.
Additionally, the combination of machine learning algorithms and quantum computing creates new possibilities for faster pattern detection and more effective data processing. Quantum machine learning methods use the parallel processing capabilities of quantum computers to handle big datasets and enhance classification, grouping, and regression problems. Examples of these algorithms are quantum support vector machines and quantum neural networks. Researchers and practitioners can use quantum algorithms to address challenging optimization problems, improve decision-making, and get insightful knowledge from data by studying the potential of quantum computing in optimization and its integration with machine learning.
It's crucial to remember that quantum computing is still in its infancy and that quantum algorithms for optimization and machine learning are currently being used in real-world applications. Nevertheless, quantum computing's capacity to solve optimization issues and transform data analysis offers considerable promise for a variety of sectors and areas.
This section examines the relationship between quantum computing and artificial intelligence (AI), with a particular emphasis on how quantum algorithms can improve machine learning models and quicken AI operations. The limits of classical computing may be solved by quantum computing, which could also offer fresh approaches to solving complicated issues that are challenging for conventional AI algorithms. Comparing quantum machine learning algorithms to traditional machine learning techniques, certain issues can be solved more effectively. Quantum algorithms, such as quantum support vector machines (QSVM) and quantum neural networks (QNN), for instance, use the concepts of quantum physics to process and analyze data in parallel, facilitating quicker pattern detection and better classification accuracy. Large datasets can be efficiently handled by quantum machine learning algorithms, which can also extract complex patterns that may be difficult for their conventional counterparts to recognize.
Quantum computing also has the ability to speed up AI training. AI model training can benefit from the use of quantum algorithms, such as quantum approximate optimization algorithms (QAOA). The training period for sophisticated AI models is shortened by these algorithms, which take advantage of quantum parallelism to more quickly explore a broader search space and identify optimal answers. Various fields, including image recognition, natural language processing, drug discovery, and recommendation systems, could develop with the combination of quantum computing and AI. Quantum AI algorithms can open up new avenues for resolving challenging issues, enhancing precision, and enabling innovations in AI-driven applications.
However, various obstacles must be overcome before quantum computing can be widely adopted. Qubit stability and coherence, error correction, scalability, and the requirement for sophisticated quantum hardware are among the hurdles. To address these issues, ongoing research is focusing on producing more stable qubits, error-correcting codes, and scalable quantum structures. Collaborations between researchers, academia, and industry are critical for pushing quantum computing developments. Companies are investing in quantum research and development in order to overcome current constraints and pave the way for commercial quantum computing technologies. By providing unprecedented processing capacity, the future of quantum computing holds the prospect of tackling increasingly difficult problems and revolutionizing businesses.
To summarize, the transformational potential of quantum computing in the field of artificial intelligence is significant. Quantum algorithms have the potential to improve machine learning models, improve pattern detection, and speed up training procedures. While hurdles exist, continuing study, funding, and collaboration will be critical in realizing quantum computing's full promise, leading to significant advances in AI and changing different industries.
About the Creator
Mide Olanrewaju
A captivating wordsmith who fearlessly explores diverse topics, weaving compelling narratives that ignite imaginations and inspire change. Prepare to be captivated by the power of their words. #Writer #Storyteller
Comments
There are no comments for this story
Be the first to respond and start the conversation.