How can SPACE and TIME be part of the SAME THING?
Space and time are constituent elements of a singular entity.
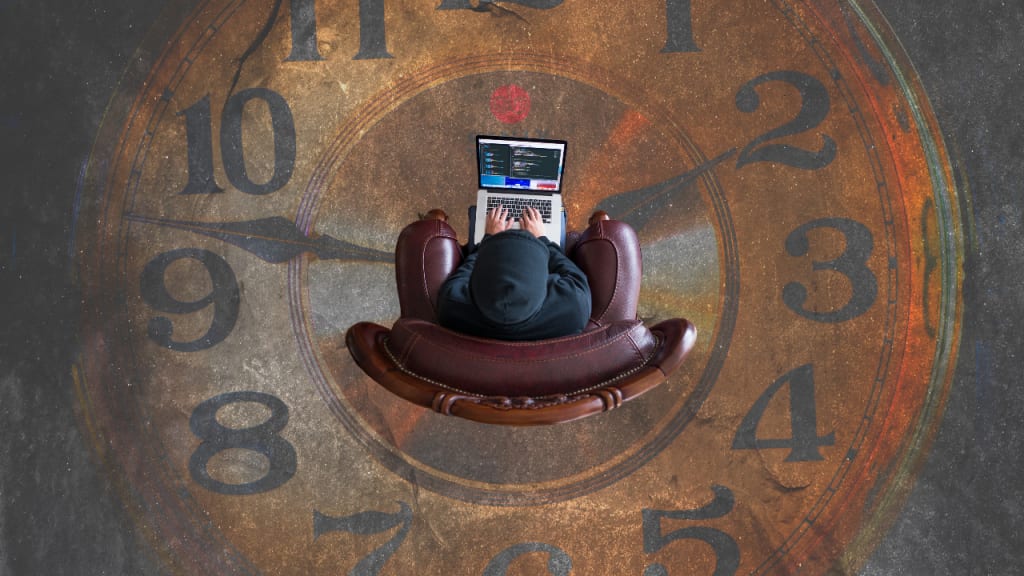
The existence of space-time is crucial for the manifestation of all known physics. Without it, there would be no platform for physical phenomena to occur. It can be likened to an artist who possesses a plethora of paint brushes in various shapes and sizes but lacks a canvas to paint on. In such a scenario, no masterpiece can be created. Similarly, without a stage, an actor is just an ordinary person. Therefore, it is imperative that physics has a place to unfold, and that place is space-time.
In the past, space was considered to be a void, an empty vacuum without any matter. However, in 1908, Hermann Minkowski proposed that time could be regarded as a fourth dimension, alongside the three dimensions of space. Later, Albert Einstein demonstrated that space-time is a form of geometry that can bend and distort, influencing the trajectory and duration of objects. Despite its omnipresence, we often take space-time for granted, much like a fish takes water for granted. But what exactly is this fundamental existential canvas that underpins the universe and our existence? How can space and time be part of the same canvas? While space is measured in meters and feet, time is measured in seconds and minutes. The apparent interchangeability of the two seems nonsensical. Why do we have only one dimension of time and three dimensions of space? Why not two, four, or five spatial dimensions and more than one-time dimension? In this article, we will attempt to provide an intuitive explanation.
The definition of space-time encompasses the entirety of points in space and time, which are identified by four numerical values. These values correspond to the location in three-dimensional space, namely x, y, and z, as well as time. These points are commonly referred to as "events". For instance, if an individual were to arrange a meeting with another person, they might specify the location as "the building situated on the corner of Fifth Avenue and Broadway, on the sixth floor", along with the designated time. Failure to provide the latter would result in the meeting being unsuccessful.
While comprehending the nature of space and time is not a challenge, as we can intuitively grasp the three dimensions of space and the passage of time, the difficulty lies in understanding how these two seemingly disparate concepts can be amalgamated into a four-dimensional continuum known as space-time. This is not an intuitive concept, as space is measured in meters and feet, while time is measured in seconds and minutes. These two units of measurement appear to be entirely distinct. The question arises as to how distance and time can be interchangeable and how they can both be components of the same entity. To gain an intuitive understanding of this question, it is helpful to compare the geometry of space and time with the geometry of space, as the latter is something we encounter daily and can relate to.
For the sake of simplicity, let us consider only two spatial dimensions. It should be noted that the concepts presented here also apply to three dimensions, although the mathematical calculations become more intricate in ordinary Euclidean space. Determining the distance between two points, A and B, is relatively straightforward. All that is required is knowledge of the coordinates in the x and y directions. With this information, we can employ the Pythagorean theorem to perform basic calculations: x^2 + y^2 = Z^2. The distance Z represents the shortest path from A to B, and it is evident that any alternative path from A to B will be longer. For instance, if we were to take a curved or zigzag path from A to B, that path would be greater in length than Z.
Now, let us examine space-time in order to establish a similarity to the 2D model. Once again, we will utilize two dimensions, but this time, one dimension will represent spatial dimension x, while the other will represent time, t. In this scenario, A and B are not solely points in space, but also in time. They can be conceptualized as events rather than mere points. The mathematical framework employed here is not based on Euclidean geometry, but rather on Minkowskian geometry. Minkowski and Euclidean geometry exhibit significant disparities. The line connecting A and B does not signify distance, but rather the time elapsed between the two events. We shall refer to this elapsed time as E - a straight line that represents travel at a constant velocity between the two events. To determine the time elapsed, it suffices to subtract the time of departure from A from the time of arrival at B. This is where the concepts of space-time and special relativity take an unintuitive turn.
One may inquire as to the distinction between the time durations of E, the elapsed time, and t, the time coordinate. E represents the time measured by an individual travelling from point A to point B, while t denotes the time coordinate of the system, akin to a universal time. Although this universal time is a human invention and does not truly exist, it can be envisioned as the time measured by an individual at rest in the universe. In the realm of Newtonian physics, prior to the advent of relativity, t and E were believed to be identical, reflecting the outdated concept of absolute time. However, Albert Einstein demonstrated that absolute time is a fallacy, leading to a new formulation.
A significant query that may arise pertains to the addition of time and distance, given that their units are disparate. The answer is that one cannot simply subtract the time of departure from the time of arrival to determine the elapsed time, as time is relative. Unlike Newton, who espoused the notion of absolute time, Einstein revealed that time is not absolute and that the ticks of a clock vary with velocity. Time flows differently for an individual in motion compared to one at rest. Consequently, the elapsed time will differ when travelling from A to B, as it necessitates a velocity in space. If an individual were stationary, moving solely through time and not space, then the time difference between A and B could be subtracted to obtain the elapsed time. However, this is not the case when travelling through space.
In order to travel from point A to point B, it is reasonable to assume that the Pythagorean theorem would not be applicable. Therefore, the equation x^2 + t^2 ≠ E^2 holds true. The correct formula in this scenario is only slightly different: E^2 = t^2 − x^2. The presence of the minus sign significantly alters the outcome. Essentially, this formula demonstrates that as one travels further in space (represented by x), the amount of time elapsed becomes shorter. There appears to be an inverse relationship between time and space. While in Euclidean space, a straight line is the shortest distance, in Minkowski’s space-time, the line connecting points A and B represents the longest duration. The more one moves in space, the less one moves in time, and vice versa. For instance, in space-time, if one were to take a curved or zigzag path from event A to event B, the elapsed time would be lower compared to a straight line between the two points. This is because more distance would have been covered in space than in time. This concept is evident in the earlier equation, but can also be illustrated through a simple example. Consider having a twin sibling who remains on Earth while you travel a significant distance in space at a certain speed and then return to Earth. Due to having travelled more in space during the same period of time that your twin remained on Earth, you would have aged less. Therefore, less time would have elapsed for you compared to your twin.
You may have a multitude of inquiries to pose. Let us address some of the most prevalent ones. Firstly, you may inquire as to the origin of this formula. This formula is derived from Minkowski's work, specifically for two-dimensional space, and the mathematical concepts involved are not overly complex. The most pressing question you may have is how to combine time and distance, given that they are measured in different units; meters versus seconds. The solution lies in a crucial conversion factor between time and space, which enables us to convert one to the other. This conversion factor is the maximum speed limit of the universe, which, to the best of our knowledge, is the speed of light. The speed of light is the fundamental element that unites space and time. In physics, we refer to this maximum speed as "c," which is equivalent to 299,792,458 meters per second. As you are aware, speed is calculated by dividing distance by time. By multiplying this speed by time, we obtain a distance, which we can express as d = c * t. Therefore, the formula operates by utilizing this conversion factor. It is worth noting that this speed can also be expressed more conveniently as one light-second per second. Consequently, converting all distances to light-seconds, rather than meters, can simplify the mathematical calculations when using seconds and meters as our units.
This straightforward formulation for a two-dimensional space-time can be expanded to encompass the genuine four-dimensional space-time in which we reside. Moreover, the foundation for comprehending General Relativity lies in 4D geometry, with the added notion that space-time is no longer flat, but rather capable of curving and contorting. The mathematical intricacies in General Relativity become quite complex rather swiftly. One of the consequences of a curving space-time is the emergence of the phenomenon of gravity. Gravity also leads to time dilation, whereby time elapses slightly slower for objects in close proximity to massive bodies such as the Earth, where gravity is more pronounced, in comparison to those situated far away in space. However, the time dilation effect resulting from gravity is less pronounced on relatively less massive objects like the Earth, in contrast to the time dilation effect caused by high velocities.
Space-time is a fundamental construct encompassing four dimensions, serving as the backdrop for the unfolding of physical phenomena, and bearing significant existential implications. One may inquire as to why precisely three spatial dimensions are present, rather than four, five, six, or more. Furthermore, the question arises as to why there is not more than one dimension of time. The absence of additional large spatial dimensions can be attributed to the fact that their existence would have been detected through empirical observation. Conversely, the inclusion of multiple time dimensions could potentially lead to the emergence of Closed Time-like Loops, enabling travel into the past. However, such a scenario is deemed implausible due to its inherent violation of causality. To illustrate, it would permit one to journey back in time and eliminate their own progenitor, thereby eradicating their own existence. This would engender paradoxes, rendering such a possibility untenable. Additionally, research indicates that any spatial dimensions beyond the four we inhabit would either render life nonviable or result in an unstable universe. Without at least one dimension of time, the absence of evolutionary progression and the absence of change would preclude the existence of life. Thus, the presence of a time dimension is indispensable. What if we were to consider the possibility of having more than three large spatial dimensions? It is worth noting that such a scenario would likely have dire consequences. In a paper published in 1917, the Austrian physicist Paul Ehrenfest demonstrated that the introduction of even a single additional dimension would result in a significant decrease in gravity, by a factor of eight, while simultaneously doubling the distance between a planet and its star. Consequently, even the slightest perturbation in a planet's orbit would lead to either its expulsion from the solar system or a catastrophic collision with the star. Furthermore, other issues have been identified, such as the instability of the electron's orbit within atoms. Conversely, if we were to have fewer spatial dimensions, the structure of space-time would be too simplistic to support life. For instance, in a universe with only one spatial dimension, the formation of stable orbits would be impossible. Similarly, a two-dimensional universe would likely lack the complexity necessary for life to emerge.
This line of reasoning leads us to the conclusion that, although it may appear anthropocentric in nature, the composition of space-time must consist of precisely three spatial dimensions, in addition to one dimension of time. Any deviation from this configuration would render our existence impossible. Therefore, we should consider ourselves fortunate to inhabit a universe that aligns precisely with these parameters.
About the Creator
Kipkemoi Dennis Ruto
In three succinct terms, I am a physicist with a penchant for writing. My written works are characterized by their ability to convey complex scientific concepts in a manner that is accessible to a broad audience.
Comments
There are no comments for this story
Be the first to respond and start the conversation.